Received 18 November 2020; Revised 11 March 2021; Accepted 14 April 2021; Published 20 May 2021
Gessynger Morais-Silva, Pedro B. Portilho, Juliana Fernandes-Santos, Rafaella M. Queiroz, Simone R. Deconte, Fernanda de A. Araújo, and Marcelo T. Marin, Interaction Between Social Defeat Stress and Chronic Ethanol Exposure on Behaviors During Ethanol Withdrawal and Pro-Inflammatory Cytokines in Mice, Psychoneuroimmunology Journal, 2 (2021), art246113. doi:10.32371/pnij/246113
Stress and ethanol consumption are known to affect the immune system, interfering in individual health, and resistance to opportunistic diseases. When experienced together, stress and ethanol seem to interact uniquely, altering each other effects depending on the exposure schedule. However, the effects of social stress on chronic ethanol exposure effects on the immune system are not well elucidated. To study this interaction, we chronically treated male swiss mice with ethanol after repeated social defeat stress (SDS) exposure. Some animals were tested in the elevated plus-maze (EPM) and open field (OF) while another cohort had plasmatic TNF-α, TGF-β1, CXCL-1, CCL-2, and VEGF quantified. Mice exposed to SDS or ethanol withdrawal showed increased anxiety-like behaviors in the EPM, while ethanol withdrawal decreased locomotor activity. Both alterations were attenuated in the groups exposed to SDS and ethanol withdrawal. Ethanol and stress alone increased plasmatic CXCL-1, while only stress affected CCL-2 and VEGF. Our results suggest that SDS exposure and ethanol withdrawal attenuated the increase in anxiety-like behaviors, but did not alter each other effects on cytokine content.
social defeat stress; ethanol withdrawal; inflammatory mediators; CXCL-1; CCL-2; VEGF; addiction
Directly or indirectly, alcohol abuse causes approximately 3 million deaths every year worldwide. Data from the World Health Organization (WHO) revealed that alcohol consumption has an annual prevalence of 43% worldwide, which is linked to several injuries and diseases. Among those alcohol-linked injuries, alcohol use disorders are responsible for most of all disability-adjusted life years (DALYs) attributable to alcohol. Diseases linked to stress and immunological alterations, as digestive diseases, cardiovascular and metabolic syndromes, infectious diseases, and malignant neoplasms contribute to more than 60% of alcohol-related deaths [1], highlighting the interaction between stress, the immune system, and alcohol consumption [2,3].
Acute and chronic stressors have an important role in the motivation for the abusive consumption of drugs [4].
However, this association is quite complex since ethanol activates brain stress-responsive systems, and in turn, the abnormal brain stress-responsive systems contribute to the
development of alcohol use disorder and relapse [5]. Stress exposure previously to ethanol administration has differential effects on ethanol effects, depending on the animal strain used, stressor type, duration, and how ethanol effects were accessed. For example, after forced-swim stress, DBA/2J and BALB/c mice show decreased ethanol intake, while no effects are seen in the C57BL/6J strain [6]. However, in C57BL/6J mice with a preexisting history of ethanol exposure, the same stressor increases ethanol consumption [7]. Ethanol administration prevents stress-induced memory impairment [8] as well as stress exposure prevents memory deficits induced by chronic ethanol consumption [9]. Both factors interact uniquely since concomitant restraint stress exposure and ethanol administration induce a specific pattern of gene expression in the hippocampus, which differs from stress or ethanol effects alone [10]. Also, despite its anxiolytic and stress-relieving effects [11], ethanol directly activates the stress-response systems [12,13].
The profile of plasmatic inflammatory mediators have been studied as possible biomarkers for alcohol abuse, as well as their role in the development and aggravation of central nervous system alterations and peripheral organ damage induced by chronic alcohol intake. Increased chemokine C-C motif ligand 2 (CCL-2) concentration, for example, is reported in the plasma and cerebrospinal fluid of alcoholics independent of the history of liver diseases [14,15,16,17], while tumor necrosis factor-alpha (TNF-α) and chemokine C-X-C motif ligand 1 (CXCL-1) are elevated in the plasma of alcoholics with liver diseases [15, 18]. On the other hand, lower levels of the transforming growth factor-beta 1 (TGF-β1) were reported in the plasma of alcoholics [14]. Similarly to ethanol effects, stress exposure also provokes diverse changes in the immune system and inflammatory mediators [19]. Indeed, some studies have linked changes in inflammatory signaling to the development of stress-related diseases [20,21,22].
Besides the great number of studies showing immunological alterations due to stress and alcohol exposure, the literature is scarce regarding the effects of their combination. Thus, our study aimed to evaluate the interactions between social defeat stress (SDS) and chronic ethanol intake on anxiety-like behavioral alterations and in the plasmatic concentration of some inflammatory mediators.
Sixty-nine male Swiss mice [Vallée Institute, Uberlândia, MG, Brazil; 7 weeks old (35–40 g) at the beginning of the experiments] were transferred to our animal facility at least five days before the beginning of the experiments and used as experimental subjects. Thirty-five mice were used for the evaluation of ethanol withdrawal and 34 mice were used to organ weighing and plasmatic cytokines quantification (Figure 1). They were housed in groups of 4 to 5 per cage, with access to food and water ad libitum (except during the chronic ethanol administration procedure) and maintained in a room at 23 ± 2 °C on a 12-h light-dark cycle. Experiments were carried out during the light phase of the cycle, under the illumination of a 100W light bulb (100 lux in the center of the apparatuses). Animals were tested randomly across this period.
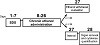
Figure 1: Procedural timeline for repeated social defeat stress and chronic ethanol administration.
All the procedures involving animal utilization were approved by the Ethics Committee for Animal Utilization of the Federal University of Uberlandia (CEUA 060/12). Experiments were conducted according to the principles of the Brazilian National Council for Animal Experiments Control (CONCEA), which are based on the NIH Guidelines for the Care and Use of Laboratory Animals.
The SDS procedure used was the resident-intruder paradigm, which is ethologically relevant and has similarities to the psychosocial stress experienced by humans. It consists of the exposure of the experimental animals to be stressed (named intruders) in the home-cage of an isolated aggressive co-specific (named resident) [23]. The residents were adult male Swiss mice [> 10 weeks old (> 40 g) during the defeats] previously selected based on their aggressiveness history and kept in unclean cages for at least two days before each encounter. Ten residents were used throughout the experiments, which were individually housed and maintained in a room at 23 ± 2 °C on a 12-h light-dark cycle, with access to food and water ad libitum.
The intruders were exposed to the resident’s home-cage for 15 min to be defeated every other day for 7 days. In the first 5 min, the intruder was placed in the home-cage of the resident protected by a protective cage, for initial recognition. After that, the intruder was removed from the protective cage and placed in confrontation with the resident mouse for 5 min or until the detection of any physical damage. Thereafter, the intruder was placed again in the home-cage of the resident protected by a protective cage for 5 min. At the end of each defeat, the intruders were placed back in their home cages until the next encounter. Each intruder was exposed to a different resident in each aggressive encounter. Body weight was measured right before the first encounter and after the last SDS exposure. Control animals were kept undisturbed in their home-cages, except for cleaning and weighting.
Chronic ethanol administration started 24 h after SDS. Ethanol 4% (v/v) in tap water was offered as the only source of water for 15 days, distributed in three cycles of 5 days, with a 2-day interval (when mice received tap water). This schedule was used to mimic a kindling mechanism in alcohol withdrawal, in which repeated experiences of withdrawal intensify its symptoms [24]. The ethanol solution was prepared fresh every day and presented to animals at the same time. Daily, when the fresh ethanol solution was prepared, the animals received an i.p. injection of ethanol (20% v/v in physiological saline) at a dose of 1.6 g/kg. Food was available ad libitum during this experiment. Body weight was measured right before the beginning of ethanol exposure and at the end of every week of ethanol treatment. Control groups received tap water and i.p. physiological saline administration.
The use of ethanol solution as the only source of water and daily i.p. ethanol injections intended to replicate episodes of high blood ethanol concentrations together with periods of extended ethanol intoxication throughout the day. After the last day of ethanol administration, animals were divided into two groups: 35 animals were used for the evaluation of anxiety-like behavior in the EPM and locomotor activity in the OF, and 34 animals were used for organ weight measurement and plasmatic cytokines quantification (Figure 1).
Ethanol withdrawal was evaluated by behavioral analyses in the elevated plus maze (EPM) and open field (OF) as already described with minor modifications [25]. Behavioral tests were carried out 22 h and 24 h after the last i.p. ethanol administration and removal of the bottles of ethanol solution, respectively.
The EPM (custom made) is a plus-shaped wooden apparatus (38.5 cm above floor level) with two open arms (30 cm length × 5 cm width) and two closed arms (30 cm length × 5 cm width, 15 cm walls) connected by a common central platform (square of 5 cm width). Twenty-two hours after the last i.p. ethanol administration and the removal of the bottle of ethanol solution, mice were placed in the central platform of the EPM facing an open arm and had their behavior recorded for 5 min by a camera fixed to the roof for analyses of the spatiotemporal and ethological variables [25]. Due to the importance of thigmotaxis to the exploration of EPM, the ethological variables head dips (exploratory movement in which animals project their heads over the edge of the open arms, HD) and stretch attend posture (risk assessment behavior in which animals stretch their body forward and then retract to the original position, SAP) were also analyzed according to the mice’s position in the apparatus, with the closed arms and central platform considered as protected areas and open arms considered as unprotected areas.
Two hours later (24 h after the last i.p. ethanol administration and the removal of the bottle of ethanol solution) mice were placed in the periphery of the OF and had their behavior recorded during 5 min for analyses of the percentage of time spent in the center, the distance traveled in the central zone, the latency to the first entry in the center and the total distance traveled [25]. The OF is a circular arena (Insight Ltd., Ribeirão Preto, SP, Brazil) of 30 cm diameter, surrounded by transparent walls (30 cm height) with its circular floor virtually divided into a central area (33% of the total area of the apparatus) and a peripheral area.
Behavioral analyses were carried out using the behavioral analysis software ANY-maze (Stoelting Co., Wood Dale, IL, USA).
Forty-eight hours after the last i.p. ethanol administration and removal of the ethanol bottles, the animals were weighted, decapitated and thymus, spleen, and adrenal glands were rapidly dissected, trimmed of fat, and weighted. Organ wet weight was expressed relative to the animal’s body weight (grams per kilogram).
Trunk blood samples were collected in heparinized tubes after decapitation, centrifuged at 9,000 g at 4 °C for 10min. Plasma was collected and kept frozen at −80 °C until the plasmatic cytokines quantification assays. TNF-α, TGF-β1, CXCL-1, CCL-2, and the vascular endothelial growth factor (VEGF) were quantified in plasma samples by immunoassay kits (R&D Systems, Minneapolis, MN, USA) following the manufacturer’s protocol. Only five animals of each group were used in plasmatic cytokines quantification. To avoid any experimenter interference, the animals were randomly chosen.
Statistical analysis was performed using Statistica software (StatSoft Inc., Tulsa, OK, USA) and graphs were made using GraphPad Prism 5 software (GraphPad Software Inc., La Jolla, CA, USA). All values were expressed as mean ± SE. The results were analyzed by two-way analysis of variance (ANOVA) considering the factors ethanol (ethanol × vehicle) and stress (stress × control). In cases where ANOVA showed significant differences (P ≤ .05), the Duncan post hoc test was performed.
Body weight gain was calculated from the first and last days of the SDS and chronic ethanol exposure (final body weight—initial body weight). Body weight gain was affected by SDS. The two-way ANOVA showed a significant effect for stress factor (F1,65 = 11.3, P < .05) but not for ethanol factor or their interaction, showing that stressed groups gain less weight when compared to nonstressed groups (Table 1). Chronic ethanol administration did not significantly affect the animal’s body weight gain (P > .1) (Table 1).

Table 1: Body weight gain of the animals during the social defeat stress exposure or the chronic ethanol administration.
Fluid intake and ethanol consumed over the chronic ethanol administration are shown in Table 2. Importantly, during chronic ethanol administration, mice were housed in groups. Thus, fluid intake and ethanol consumed during this period cannot reflect individual differences in drinking, but provide an estimative that fluid intake and ethanol consumed were similar between the groups.

Table 2: Fluid and ethanol consumed over the chronic ethanol administration procedure.
Behavioral analyses in the EPM showed an increase in anxiety like-behavior in mice exposed to SDS or chronic ethanol administration, while the SDS pre-exposure attenuated the increase in anxiety like-behavior induced by ethanol withdrawal (Figure 2).
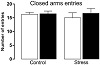
Figure 2: Spatial and ethological behaviors in the elevated plus-maze of mice exposed to chronic social defeat stress and ethanol administration. The test was performed for 5 min after 22 h of ethanol withdrawal. (a) Number of entries in the closed arms; (b) percentual number of entries in the open arms; (c) percentage of time spent in the open arms; (d) number of head dips performed; (e) percentage of head dips performed in the protected areas of the apparatus; (f) number of stretches performed; (g) percentage of stretch attend postures performed in the protected areas of the apparatus. Data are reported as mean ± SE (n = 8–9 animals per group). a, P < .05 relative to control-vehicle group; d, P < .05 relative to stress-ethanol group.
Closed arms entries (Figure 2(a)) were not affected by the treatments. The two-way ANOVA did not show any significant effect regarding closed arms entries (P > .1).
The two-way ANOVA for the open arms entries (%) (Figure 2(b)) showed significant effect for the interaction between stress and ethanol factors (F1,31 = 9.32, P < .01) with Duncan post hoc test revealing a decrease in the percentage of open arms entries in control/ethanol and stress/vehicle groups when compared to control/vehicle (control/vehicle × control/ethanol, P = .05; control/vehicle × stress/vehicle, P = .05) and stress/ethanol group (stress/ethanol × control/ethanol, P < .05; stress/ethanol × stress/vehicle, P < .05). A decrease in the time spent in open arms (%) (Figure 2(c)) was also found in control/ethanol group. The two-way ANOVA showed significant effect for the interaction between stress and ethanol factors (F1,31 = 5.81, P < .05). The Duncan post hoc test revealed a decrease in the percentage of time spent in open arms in control/ethanol group when compared to control/vehicle group (P = .05) and a trend of reduction in stress/vehicle group when compared to control/vehicle group (P = .07).
Regarding to the HD (Figure 2(d)), the two-way ANOVA showed significant effect for the stress factor (F1,31 = 6.26, P < .05) and for the interaction between stress and ethanol factors (F1,31 = 17.36, P < .001), with control/ethanol, stress/vehicle and stress/ethanol groups showing a reduction in the number of HD when compared to control/vehicle group (P < .01, P < .001, P < .05, respectively). The stress/vehicle group also showed a reduced number of HD when compared to the stress/ethanol group (P < .05). When HD was analyzed in the protected zone of the apparatus (Figure 2(e)), the control/ethanol group showed an increase in expression of this behavior. The two-way ANOVA showed a significant effect for the interaction between stress and ethanol factors (F1,31 = 4.06, P = .05) with Duncan post hoc test revealing an increase in the percentage of protected HD in control/ethanol group when compared to control/vehicle group (P < .05).
Regarding to SAP (Figure 2(f)), the two-way ANOVA showed significant effect for the ethanol factor (F1,31 = 8.22, P < .01) and for the interaction between stress and ethanol factors (F1,31 = 12.94, P < .01). The Duncan post hoc test revealed an increase in SAP in the groups
control/ethanol, stress/vehicle and stress/ethanol when compared to control/vehicle group (P < .001, P < .01, P < .05, respectively). Similar results were found when SAP was analyzed in protected zone of EPM (Figure 2(g)). The two-way ANOVA showed significant effect for ethanol factor (F1,31 = 5.24, P < .05) and for the interaction between stress and ethanol factors (F1,31 = 6.59, P < .05). The Duncan post hoc test revealed an increase in protected SAP in the groups control/ethanol, stress/vehicle and stress/ethanol when compared to control/vehicle group (P < .01, P < .01, P < .01, respectively).
The two-way ANOVA showed a significant effect for the interaction between stress and ethanol factors (F1,31 = 8.63, P < .05) on total distance traveled in the OF (Figure 3(a)). Duncan’s post hoc test revealed a reduction in the total distance traveled in the group control/ethanol when compared to control/vehicle and stress/ethanol (P < .01, P < .05, respectively).
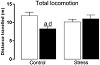
Figure 3: Locomotor activity in the open field apparatus of mice exposed to chronic social defeat stress and ethanol administration. The test was performed for 5 min after 24 h of ethanol withdrawal. (a) Total distance traveled in the apparatus; (b) latency to enter for the first time in the center of the apparatus; (c) time spent in the center of the apparatus; (d) distance traveled in the center of the apparatus. Data are reported as mean ± SE (n = 8–9 animals per group). a, P < .05 relative to control-vehicle group; b, P < .05 relative to control-ethanol group; d, P < .05 relative to stress-ethanol group.
The latency to enter in the center of the OF (Figure 3(b)) was also altered by the treatment. The two-way ANOVA showed a significant effect for the interaction between stress and ethanol factors (F1,29 = 10.05, P < .01). Duncan post hoc test revealed an increase in the time necessary for the first entrance in the center of the OF in stress/vehicle group relative to all the other groups (stress/vehicle × control/vehicle, P < .01; stress/vehicle × control/ethanol, P < .05; stress/vehicle × stress/ethanol, P < .01).
No significant alterations were found neither in the time spent in the center (Figure 3(c)) nor in the central locomotion (Figure 3(d)) (P > .05).
The treatments affected adrenal and thymus, but not spleen weight. There was adrenal hypertrophy in ethanol groups, while both stress and ethanol accelerated the thymic involution (Table 3).

Table 3: Organ weight of animals exposed to social defeat stress and chronic ethanol administration.
Two-way ANOVA showed a significant effect for ethanol factor altering the adrenal absolute (F1,30 = 7.00, P < .01) and relative weight (F1,30 = 7.26, P < .01). Regarding to thymus absolute weight, the two-way ANOVA showed a significant effect for the interaction between stress and ethanol factors (F1,29 = 4.83, P < .05). Duncan post hoc test revealed a significant decrease in control/ethanol, stress/vehicle and stress/ethanol groups when compared to control/vehicle group (P < .05). The two-way ANOVA showed a significant effect for ethanol factor (F1,29 = 7.22, P < .01) and for the interaction between stress and ethanol factor (F1,29 = 4.53, P < .05) altering the thymic relative weight. Duncan post hoc test revealed a significant decrease in control/ethanol, stress/vehicle and stress/ethanol groups when compared to control/vehicle group (P < .01).
No significant alterations were found neither in absolute nor in relative spleen weight (P > .05).
Ethanol and stress treatments increased plasmatic CXCL-1, while only stress exposure affected CCL-2 and VEGF content.
No significant alterations were found neither in TNF-α (Figure 4(a)) nor in TGF-β1 (Figure 4(b)) plasmatic content (P > .05).
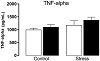
Figure 4: Plasmatic cytokines content of mice exposed to chronic social defeat stress and ethanol administration. Blood collection was performed after 48 h of ethanol withdrawal. (a) Concentration of tumor necrosis factor-alpha (TNF-α, pg/mL) in plasma; (b) concentration of the transforming growth factor-beta 1 (TGF-β1, pg/mL) in plasma; (c) concentration of the C-X-C motif chemokine ligand 1 (CXCL-1, pg/mL) in plasma; (d) concentration of C-C motif chemokine ligand 2 (CCL-2, pg/mL) in plasma; (e) concentration of vascular endothelial growth factor (VEGF, pg/mL) in plasma. Data are reported as mean ± SE (n = 5 animals per group). a, P < .05 relative to control-vehicle group; b, P < .05 relative to control-ethanol group; ∗, overall stress effect (P < .05).
The two-way ANOVA showed a significant effect for stress factor (F1,16 = 32.25, P < .001) and a trend for the interaction between stress and ethanol factors (F1,16 = 3.15, P = .09) regarding to CXCL-1 content (Figure 4(c)). Duncan post hoc test revealed an increase in control/ethanol, stress/vehicle and stress/ethanol groups when compared to control/vehicle group (P < .05, P < .001, P < .001, respectively). Stress/vehicle and stress/ethanol groups CXCL-1 concentration were also increased in relation to control/ethanol group (P < .01, P < .05, respectively).
Regarding to CCL-2 (Figure 4(d)) and VEGF (Figure 4(e)), the two-way ANOVA revealed a significant effect only for stress factor, showing an increase in CCL-2 plasmatic concentration (F1,16 = 8.40, P < .05) and a decrease in VEGF plasmatic concentration (F1,16 = 16.29, P < .001).
Our findings showed that despite the anxiogenic effect of the SDS exposure or ethanol-withdrawal, the animals exposed to both SDS and ethanol administration showed attenuated anxiety-like behaviors. On the other hand, previous SDS exposure did not alter adrenal hypertrophy, thymic atrophy, or CXCL-1 content increase induced by chronic ethanol administration and withdrawal. Ethanol administration after SDS also did not alter stress-induced alterations in thymic atrophy, CCL-2, and VEGF content.
The EPM and the OF tests are widely used for the evaluation of anxiety and locomotor activity in rodents. Both models are highly ethologically relevant to the species in which they are performed and are sensitive to clinically used anxiolytic drugs [26]. More importantly, they are broadly used and validated to study the consequences of stress and drug exposure [27,28], and complement each other in the evaluation of the rodents’ defensive behavioral repertoire [29]. The EPM was developed to study anxiety in rodents based on the natural aversion of such species to open and elevated spaces, using spatiotemporal measures to the evaluation of anxiety-like behaviors [30,31]. Years later, the addition of ethologically relevant behavioral endpoints, that are naturally performed by the animals in their natural environment, increased the robustness of the model [’]. The HD and SAP are behaviors related to the mice exploratory activity and risk assessment in the open arms of the EPM, while the protected forms (when such behaviors are performed in the closed arms or the center of the apparatus) are intrinsically related to anxiety levels. The statistical factor analysis of the mice behaviors’ in the EPM revealed that both HD and SAP were highly loaded in the risk assessment/decision-making factors, while the protected HD and SAP were highly loaded in the anxiety factor [33]. The treatment with chlordiazepoxide, a benzodiazepine anxiolytic, decreases the frequency of SAP and the protected SAP and HD, while increases the frequency of HD [34]. On the other hand, exposure to social stressors increases the frequency of SAP and protected HD [35]. The protected SAP are also correlated to the corticosterone response to the EPM [36]. In our study, we found a decrease in HD and an increase in SAP and protected SAP and HD in control/ethanol and stress/vehicle groups, in addition to decreased open arms entries and time. Thus, we could interpret those groups were presenting increased risk assessment and anxiety levels. Increased anxiety-like behaviors are commonly reported in rodents exposed to stressful situations and after the withdrawal from chronic ethanol experience [25,27,37,38].
Experiments involving one exposure to the OF are generally looking for the rodents’ reactivity to this new environment. This exposure to a new, illuminated and open space is stressful and induces a repertoire of behaviors that are related to the exploration and risk assessment of the new ambient, and, consequently, anxiety. The time and distance traveled in the center of the apparatus and the latency to enter in the center are measures used to the evaluation of anxiety in this apparatus, while total distance traveled is used as an index of general activity [39]. Here, we found an
increased latency to enter the OF center in the stress/vehicle group, while the control/ethanol group showed decreased total distance traveled, indicating an increase in anxiety-like behaviors in stressed animals and a decrease in general activity in ethanol-treated mice. Decreases in locomotor activity in the OF are often reported in rodents experiencing ethanol-withdrawal after chronic drug intake [40,41,42].
As cited before, both SDS and ethanol withdrawal are known to induce anxiogenic responses in animal models. When interacting, however, the behavioral consequences of stress and ethanol exposure seem to be more complicated. Restraint stress induces an anxious mode in alcohol-deprived rats [43] and increases the duration of withdrawal-induced emotional abnormality in mice [44]. This effect is different from those found for forced swim stress exposure, which does not alter ethanol-induced locomotor deficits during withdrawal [25], despite increasing the consumption of more concentrated ethanol solutions. Continuous SDS increases ethanol-induced conditioned place preference and blocked the ethanol-induced increase in locomotor activity [37]. The same research team showed that acute ethanol treatment does not alter SDS effects on social interaction in mice [45], while previous SDS impairs the locomotor stimulant effect of an ethanol challenge [46]. Surprisingly, in our work, although ethanol or SDS exposed animals had shown increased anxiety-like behaviors, animals from the ethanol/stress group showed attenuated anxiety-like behaviors in the EPM and OF.
It is unclear to us how SDS and alcohol-withdrawal interacted in animal’s brains in this study to prevent each other effects on anxiety-like behaviors. One possibility lies in brain limbic structures altered by ethanol or stress. Ethanol-withdrawal and stress change the activity of similar brain structures related to the encoding of motivational salience of a stimulus (i.e., mesocorticolimbic dopaminergic pathway) and emotional behavior (e.g., amygdala and other limbic structures) [4]. When experienced together, forced-swim stress and ethanol consumption increase dopaminergic content within the amygdala, abolishing drug conditioned place preference [47]. Stress induces the activation of dopamine release in medial prefrontal cortex neurons, which in turn inhibits dopamine release in the nucleus accumbens [48]. An acute dose of ethanol reverts some SDS effects on dopamine and serotonin levels in the hippocampus and frontal cortex [46]. In turn, the above-mentioned structures and neurotransmitters share an important sensitivity to the corticotrophin-releasing factor (CRF) and glucocorticoids, which are related to both stress and ethanol-withdrawal behavioral alterations [49,50]. For example, ethanol-withdrawal effects are blocked by the administration of type 1 CRF receptor (CRF-R1) antagonists [51,52]. Likewise, ethanol-withdrawal experience alters CRF and CRF-R1 immunoreactivity in animal models [53,54,55]. CRF signaling is also involved in SDS anxiogenic effects [55,56]. Thus, previous SDS exposure may have led to plastic changes in CRF signaling, which could impair ethanol effects on this neuronal system and consequently mitigate the increase in anxiety-like behaviors induced by the ethanol-withdrawal. The same logic could be applied on the contrary: experiencing ethanol-withdrawal after SDS could impair the plastic changes in CRF signaling related to SDS exposure, blocking the increase in anxiety-like behaviors related to the stress exposure. Both scenarios are hypothetic, and new experiments are necessary to confirm such possibilities.
Adrenal hypertrophy and increased thymic involution are anatomical alterations that could be interpreted as a sign of hypothalamic-pituitary-adrenals (HPA) axis chronic activation [57,58,59]. Stressful situations [58,60] and ethanol intake [12,13,61] activate hypothalamic neurons from the paraventricular nucleus to increase CRF release in the anterior pituitary, which in turn stimulates adrenocorticotropic hormone (ACTH) release to stimulate glucocorticoid production and release by the adrenals glands. Thus, in our study, stress, and ethanol do not alter each other’s effects on HPA axis activation, since both stress and ethanol-treated animals showed increased thymic involution. Regarding the stress effects on adrenal weight, it was expected an increased organ weight in the vehicle-stress group. However, organ dissection was held weeks after the last SDS exposure. At this time point, adrenal glands could have recovered from chronic stress exposure.
Although glucocorticoids are known to be potent modulators of the immune function [this modulation is elegantly reviewed in [62]], stress regulates immunological parameters through other immune modulators in addition to glucocorticoid release [63,64,65]. In this sense, there is a disconnection between the HPA axis, which shown habituation to repeated stress exposure, and immunological alterations, which are increased after chronic stress exposure [22,66,67,68]. Our results corroborate such findings since we did not find adrenal hypertrophy in stressed animals, which presented the most robust effects on cytokine profile. After 30 days of SDS, glucocorticoid-sensitive genes do not respond anymore to elevated plasmatic corticosterone in defeated mice [69]. On the other hand, increased CXCL-1 expression is found in mice exposed to chronic psychosocial stress, and it is related to the inflammatory aggravation of intestinal inflammatory diseases in this animal model [70]. Monocyte infiltration is crucial for lung metastatic colonization of circulating tumor cells that are induced by exposure to psychosocial stress. This effect is mediated by CCL-2 overexpression and Beta-adrenergic signaling [71]. This chemokine expression is also increased after chronic mild stress exposure [72] and in patients who suffered from acute coronary syndrome after emotional stressors [73].
In addition to its pro-angiogenic effect, VEGF is an important mediator of vascular relaxation in adults, through the nitric oxide signaling pathway [74]. Stressed mice in our study showed decreased VEGF content in plasma, contrary to the findings of Stewart and colleagues in 2015 [75]. Although it is difficult to explain such discrepancies, we believe that our result could be related to stress effects on blood pressure and vascular contraction, which increases the risk of the development of hypertension.
It is not yet known how ethanol influences the immune system. Alcohol intake also activates the HPA axis, leading to increased glucocorticoid release to the bloodstream. Moreover, in-vitro studies show the direct action of this drug on immune cells, and a possible role of acetaldehyde, the main ethanol metabolite [76]. Acutely, moderate ethanol intake reduces NF-κB signaling in macrophages, an important intracellular pathway related to pro-inflammatory responses [77]. On the other hand, chronic alcohol consumption increases NF-κB signaling [78] and impairs HPA axis functioning [5], in addition to increased oxidative stress. At least for alcohol-induced liver injury, CXCL-1 upregulation is an important factor in the development and aggravation of the disease [79].
Cytokines alterations found in our study seem to be related to a switch in inflammatory mediators to a proinflammatory profile. Stress exposure increased CCL-2 and CXCL-1, while withdrawal from chronic ethanol administration increased CXCL-1 concentration. CXC chemokines are important chemoattractants for neutrophils and T lymphocytes, while CC chemokines are potent attractants for monocytes, basophils, and eosinophils. Specifically, CXCL-1 is known mainly due to its effects on neutrophils, whereas CCL-2 has a potent effect on monocytes [80]. Thus, increased CXCL-1 and CCL-2 plasmatic concentration in our study could be interpreted as a factor that increases immune responses over a physiological range. An interesting feature of alcohol and stress exposure is the dual effect, depending on the time and intensity of the event. Acute stress and alcohol intake have anti-inflammatory properties, while chronically they increase pro-inflammatory pathways [19,76].
Our results suggest that stress and ethanol interaction do not occur in the HPA axis. It is evidenced by the lack of alteration in corticosterone-related alterations in animals that were both chronically stressed and received ethanol. This data corroborates a previous study showing no alterations in corticosterone response to forced-swim stress after chronic intermittent treatment with ethanol in vapor chambers [81]. However, the time point and duration of individuals experience ethanol or stress, and protocols of stress used seem to be crucial to their interaction. Acute binge-like drinking and i.p. ethanol administration increases corticosterone response to restraint stress [82], while adrenal response to LPS-induced stress response is attenuated in animals under ethanol effect [83]. Previous experience of chronic SDS attenuates plasmatic corticosterone increase induced by an acute ethanol challenge [46]. In humans, moderate alcohol consumption after stress exposure facilitates the recovery of the endocrine responses to stress [11]. Chronic ethanol treatment increases corticosterone response to immobility stress [84] but attenuates corticosterone response to LPS [85]. Thus, more studies are necessary to elucidate how stress and ethanol interact regarding the HPA axis activation and their effects on the immune system.
Although this study did not use females in the experiments, there is evidence for sex differences for both stress and alcohol experience effects. Women are more vulnerable to stress-related mood disorders [86,87] while men are more likely to develop substance abuse problems [87]. Also, studies suggest that women have a pro-inflammatory immunological profile compared to men [88], probably due to the strong immunosuppressant effects of testosterone [89]. On the other hand, after stress exposure, females showed an anti-inflammatory response, while pro-inflammatory pathways are increased in males [90,91]. Binge drinking in adolescence increases the levels of inflammatory cytokines in females, but not males [92].
In conclusion, SDS exposure followed by chronic ethanol administration and withdrawal experience attenuates the increase in anxiety-like behaviors related to ethanol-withdrawal or SDS exposure but has no effects on the physiological consequences of chronic ethanol consumption or SDS experience in the immune system. Stress exposure increases the plasmatic content of CXCL-1 and CCL-2 cytokines and decreases VEGF plasmatic concentration, while ethanol experience increased CXCL-1 concentration. Such alterations could be related to their aggravating effects on inflammation-related diseases.
This work was supported by Fundação de Amparo à Pesquisa do Estado de Minas Gerais (FAPEMIG) (CBB-APQ-04545-10).
The authors declare that they have no conflict of interest.
Copyright © 2021 Gessynger Morais-Silva et al. This is an open access article distributed under the terms of the Creative Commons Attribution License, which permits unrestricted use, distribution, and reproduction in any medium, provided the original work is properly cited.