Vol. 7 (2024), Article ID 246164, 8 pages
Research Article
Design and Construction of a Novel Green Optode for Hafnium Determination in Real Environmental Samples
Abdullah A. A. Sari,1 K. F. Dbbabi,1,2 Refat El-Sayed,1,3 Alaa S. Amin,3 and Eslam M. I. Moustafa3
1Department of Chemistry, University College in Al-Jamoum, Umm Al-Qura University, Makkah 21955, Saudi Arabia
2Department of Chemistry, Higher Institute of Applied Sciences and Technology of Mahdia, University of Monastir, Monastir, Tunisia
3Chemistry Department, Faculty of Science, Benha University, Benha, Egypt
Received 20 December 2023; Revised 31 March 2024; Accepted 17 April 2024; Published 17 December 2024
Abdullah A. A. Sari, K. F. Dbbabi, Refat El-Sayed, Alaa S. Amin, and Eslam M. I. Moustafa, Design and Construction of a Novel Green Optode for Hafnium Determination in Real Environmental Samples, Journal of Transition Metal Complexes, 7 (2024), art246164. doi:10.32371/jtmc/246164
A highly selective and sensitive Hf optode was developed, which uses a triacetylcellulose membrane to immobilize 4-(2-benzothiazolylazo)2,3-biphenyldiol (BTABPD). The membrane optode responds to hafnium ions by color changing reversibly from yellow-orange to pink in thiel buffer at pH 5.0. The proposed membrane under optimum experimental conditions exhibits a linear dynamic range of 5.0–185 ng cm−3 of the Hf(IV) with detection and quantification limits of 1.58 and 4.95 ng cm−3, respectively. The newly developed optode has a response time of 15–30 s based on the Hf4+ ion level. The optode’s response is determined by the pH range of 4.5–5.5. The complex’s quantification and detection limits, Sandell sensitivity, molar absorptivity, Ringbom, calibration ranges, and relative standard deviation (RSD) have all been accurately calculated. The presented optode records fast response time, low detection limit, and, most significantly, excellent selectivity towards a number of lanthanide and transition ions (Sc3+, Y3+, La3+, Ce3+, Gd3+, Sm3+, Lu3+, Tm3+, Ho3+, Dy3+, Eu3+, Cd2+, Cu2+, Ni2+, Co2+, Pb2+, Bi3+, Al3+, Sn2+, Mn2+, Tl+, Ag+, Zn2+, Fe2+, Cr3+, and Zr4+ ions). The optode was regenerated successfully with thiourea and its response was reproducible (RSD ≤ 1.9%) and reversible. The proposed optode was successfully applied to detect Hf(IV) ions in real samples.
optode; hafnium determination; 4-(2-benzothiazolylazo)2,3-biphenyldiol; triacetylcellulose; environmental analysis
The primary contaminant in zirconium compounds, which have a 2.0–3.0% Hf(IV) content, is hafnium(IV). Both hafnium and zirconium, if applied in the nuclear industry, must be separated. Hafnium absorbs neutrons much better than any other element and is resistant to corrosion; therefore, it is used to control nuclear reactions. Hafnium is applied for alloying with aluminium, iron, titanium, and other elements. It is resistant to corrosion because it is an impenetrable oxide layer on its surface and hard. Hafnium is utilized in high-temperature alloys in the ceramics sector because of its extremely high melting point [1,2].
The most methods often used for the above purpose are isotope dilution neutron activation analysis (IDNAA) [3,4], neutron activation [5], X-ray fluorescence [6], and isotope dilution mass spectrometry [7] used as standard natural hafnium solutions [1,2,3]. These procedures are usually free of interference from diverse metals [5]. Nevertheless, to counteract or minimize the influence of the matrix on the detection of the micro-component, hafnium, other methods of Hf(IV) detection require the preparation of standards using a matrix consisting of extremely pure zirconium (zirconium salts).
From an analytical perspective, it is crucial to identify Hf ions in alloys and other substances directly. Developing a highly selective and sensitive spectro-photometric method for Hf4+ is crucial. To increase sensitivity, inductively coupled plasma atomic emission spectrometry and electrothermal atomic absorption spectrometry were employed; however, these techniques typically need costly and time-consuming equipment, which is only available in some facilities [8,9]. These days, spectrophotometric methods are among the most used ways to identify metal ions. Hf(IV) was detected spectrophotometrically using a variety of reagents [10,11,12,13,14]. A small number of chromogenic reagents however are available for the spectrophotometric determination of Hf. Alizarin red S [15], xylenol orange [16], Arsenazo III [17,18], 4-(2-thiazolylazo)resorcinol (PAR) [19], 1-(2-pyridylazo)-2-naphthalenol (PAN) [20], and methylthymol blue [21] were recommended for spectrophotometric detection of Hf(IV). These procedures have their own advantages and disadvantages. The detection is interfered by various ions such as Cu2+, Co2+, Cd2+, Be2+, Hg2+, Mn2+, Pb2+, Sn2+, Fe3+, Cr3+, Zr4+, Si4+, Re4+, and W6+. The requirement to separate the various ions from the solution before Hf determination was a drawback of these methods [21].
The determination of very low concentrations of Hf usually requires separation and preconcentration steps [22,23,24]. The determination of the traces of Hf in presence of Zr is very difficult by classical wet chemical methods [25]. Since Zr and Hf undergo hydrolysis and polymerization in solution with other elements, consideration must be used during sample decomposition and dissolution for these analyses. The separation of Zr and Hf is a laborious procedure because these two elements have similar chemical characteristics. Zr-Hf separation techniques include liquid-liquid extraction, ion exchange chromatography, and precipitation. Since zircon is a refractory material, quantitative dissolution requires fusion rather than acid attack [26]. Flame AAS has trouble analyzing Zr and Hf because there is not enough production of free atoms in the flame, which results in low detection limits. When zircon is dissolved using fusion mixes, the total dissolved solids increase, which causes significant issues with nebulization to ICP-OES or ICPMS [27]. By contrast, ZrO2 and HfO2 can be individually determined at wide concentrations using a technique called wavelength dispersive X-ray fluorescence spectrometry (WDXRFS). Using a quick and easy sample preparation procedure including a finely ground (<63 μm) powdered sample pellet, WDXRFS analysis yields extremely exact results in a non-destructive manner [28]. However, the use of WDXRFS requires many standard samples for reference.
Over the last three decades, the field of optical chemical sensors (optodes) has been a growing field area. Chemical sensor technology involves subsequent transduction of the analytical signals and the key processes of chemical recognition of the analytes of interest. The development of optodes [29] has been mostly dependent on the immobilization of the chromophore by either chemical (covalent bond) or physical (sol-gel, adsorption, encapsulation, etc.) procedures and incorporated in the membrane design. The immobilization process can be carried out either directly on the surface of the optical fibers (intrinsic optodes) or on the best material that can serve as a bridge between the sample and the fiber optic system (extrinsic optodes) [30]. The sensing phase consists of immobilized chromophore reagents in either inorganic or organic matrices. The absorbance behavior of the sensitive layer is changed when it reacts with the analyte. Optode design relies on metalochromic indicators and organic dyes, which are essential components for the spectrophotometric detection of various metals [31].
There is no report on the immobilization of 4-(2-benzothiazolylazo)2,3-biphenyldiol (BTABPD) on a triacetylcellulose membrane, to the best of our knowledge; BTABPD has been applied to the spectrophotometric determination of niobium(III) [32], bismuth(III) [33], titanium [34], and palladium [35]. In the present paper, an optode fabrication is described to determine ultra-trace amounts of Hf(IV) in which the sensing chromophore is BTABPD immobilized on triacetylcellulose membrane. Basic theoretical and principles description of bulk optode membranes based on the reversible mass analyte transfer from the sample in the bulk of the sensing layer have been well elucidated [30,31]. The reaction only takes a few minutes to complete, and when measured using spectroscopic detection in the absorbance mode, the color changes from yellow-orange to pink. The membrane layer can be quickly and completely refreshed using a thiourea, which makes the optode fully reversible. The results obtained indicate that the proposed optode is an effective instrument for determining
the Hf(IV) content in various environmental samples.
All chemicals and reagents were achieved from analytical reagent grade chemicals supplied from Merk (Darmstadt, Germany). A stock Hf(IV) solution was prepared by dissolving appropriate weights of HfOCl2 ⋅ 8H2O (Merck) in 100 cm3 of 0.1 mol dm−3 HCl to make a 1,000 μg cm−3 solution. A 10 μg cm−3 working standard solution was prepared by accurate dilution of the stock ones with deionized water. Thiel buffer solutions of various pH values 2–12 were prepared as represented earlier [36]. Stock solutions of 15,000 ng cm−3 of interfering ions were prepared by dissolving appropriate weights of suitable salts in deionized water. BTABPD was synthesized using conventional diazotization and coupling methods described earlier [33].
A Hamilton syringe (10 μL) was used to deliver trace volumes of Hf4+ into the cell. The reference cell contained a membrane prepared in the same way without Hf4+. A Perkin-Elmer Lambda 12 UV-vis spectrophotometer with a 10 mm quartz cell was used for all spectral measurements. All measurements were made in the λmax of 616 nm. An Orion research model 601A/digital ionalyzer pH meter was used for checking the pH of solutions. A Perkin Elmer
atomic absorption spectrometry model AAnalyst 300 was used for all GFAAS measurements.
The sensing membrane was fixed and placed in a cuvette. The length of the film is exactly as the length of the cuvette. Thus, the optode is placed in the cell and cannot move during the determinations. All measurements were performed in a batch mode. Optode membrane response to various metal ions was studied. Membrane was first exposed to buffer solution and absorbance was measured at 497 nm. Then, the sample solution was added and absorbance at 616 nm against BTABPD membrane was measured after 1 min. All results are based on six replicate measurements.
Triacetylcellulose transparent membranes were produced from waste photographic film tapes, which were previously treated with commercial sodium hypochlorite for one min in order to remove the colored gelatinous layers. Films (1 cm × 4 cm) were treated with a clear BTABPD (3 mg) in 10 mL ethylene diamine for 2 min at 25±2.0 °C. Afterwards, they were washed with deionized water for the removal of ethylene diamine and the loosely trapped chromophore (BTABPD). Membranes were finally washed with detergent solutions and water. Prepared membranes were kept under water, when not in use [37].
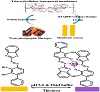
Scheme 1: Representative diagram for the preparation and complexation of the formed optode.
To reach equilibrium, the prepared optode membranes were inserted in a buffer of pH 5.0 for 3 min. Then, membranes were put vertically inside the sample and blank cuvettes containing 2.5 cm3 buffer of pH 5.0. The sample cell was finally titrated with Hf(IV) ions solution and the absorbance value was measured over the wavelength range of 400–740 nm after 3 min. The absorbance of optode membrane was measured, at λmax of 616 nm.
Real water samples containing various Hf(IV) levels were prepared by adding known amounts of Hf(IV) to the samples. The Hf(IV) content of the each samples solution was detected by the proposed optode applying the standard addition technique.
River water samples (from different locations of river, Benha, Egypt) did not require previous treatment and were analyzed for Hf4+ ion level applying the described optode.
pH of river water was adjusted by the addition of 2 cm3 of thiel buffer (pH 5.0) to 10 cm3 of river water.
In order to confirm the high usefulness and resolution of the apparatus, Hf(IV) present as an impurity in commercial high-purity zirconium oxides achieved from three various makers was determined with it. The results when compared with those obtained by ICP-AES show that the performance of the apparatus has been confirmed. In addition, it was found that the amounts of Hf(IV) contained in these so-called “high-purity” zirconium oxides are of the order expected for zirconium oxide not subjected to purification.
A 0.5 g of each sample was weighted and transferred into a 30 cm−3 platinum vessel separately to determine Hf(IV) in soil and copper-based alloy. A 10 cm−3 of concentrated HNO3 and 5 cm−3 of concentrated HCl were added into each of the vessels. Each mixture was heated until complete evaporation of its solution. This process (digestion) was replicated three times, and then 2 cm−3 of concentrated HNO3 and 5 cm−3 of concentrated HF were added into each vessel and heated to boiling point. The mixture was filtered and washed three times by 10 cm−3 deionized water. The filtrates were evaporated to a volume of 3 cm−3, and then the pH was adjusted to 5.0 by adding a 1 mol dm−3 solution of NaOH. A suitable amount of ammonium acetate salt was added so that its concentration was reached to 0.3 mol dm−3 in a final volume of 5 cm−3.
The absorption spectra of immobilized BTABPD on hydrolyzed cellulose acetate were represented in Figure 1, after equilibration in a buffer of pH = 5.0 containing various levels of Hf(IV) ions. The spectral characteristic of the BTABPD membrane indicates a maximum band that appears at 497 nm (Figure 1). The spectral change is the result of the addition of Hf(IV) and complex formation in the optode (Figure 1). The spectral change to bathochomic shift (increase in absorption band by 119 nm) is the result of the increase of Hf(IV) ions amount in the membrane, which is due to its extraction into the membrane and complex formation. For all additional studies, the λmax of 616 nm was selected, due to higher sensitivity and selectivity at this wavelength.
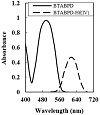
Figure 1: Absorption spectra for 100 ng cm−3 Hf(IV) complexed and immobilized BTABPD at pH 5.0.
The response of the optode was investigated through an examination of various buffer solutions. Experiments were conducted on acetate, phosphate, borate, thiel, and universal buffers. Thiel buffer solution was the best buffer. The effect of the pH value on the optode membrane’s reaction is shown in Figure 2. The absorbance measurements were made for 100 ng cm−3 Hf(IV) ions in the pH range of 2.5–12 at 616 nm. It was represented that the hafnium complex was absorbed maximally at pH 5.0. In subsequent investigations, the pH was maintained a maximum absorbance at pH 5.0. Moreover, the addition of 2.0–4.0 cm−3 of the buffer did not affect the absorbance of the complex and the use of
2.5 cm−3 is recorded. At pH values lower than 5.0, the absorbance starts to increase. This phenomenon might be due to the fact that at lower pH values (pH < 5.0), complexation is weak. At pH values higher than 5.0, Hf(IV) complex decreases gradually slowly due to the dissociation of the formed complex with increasing pH values. Thus, pH 5.0 was selected for further works.
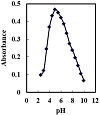
Figure 2: Absorbance of the optode in solutions containing 100 ng cm−3 Hf(IV) at different pH values.
After examining several immobilized phases, triacetyl-cellulose membrane was found to be the most effective. The response time of an optode is a crucial analytical characteristic. The optode response time is controlled by the time needed for the analyte to diffuse from the bulk to the membrane interface and to complex with the chromophore [38]. The optode response time was tested by measuring the absorbance change at 616 nm from a buffer (pH 5.0) to a buffered Hf(IV) solution of 100 ng cm−3. The membrane was found to reach 97.5% of the absorbance at 45–60 S depending on the amount of Hf(IV) ion (Figure 3). In general, the response time is lower in concentrated solutions than dilute ones due to strict hindrance in the membrane to form a complex. In general, the response time of the optode membrane is governed by three processes: (1) film diffusion, (2) complex formation rate between metal ion and ligand, and (3) complex dissociation rate [38]. It should be noted that the binding of the Hf(IV) ion to BTABPD is a kind of coordination to the OH group and the nitrogen of the azo group of the ligand.
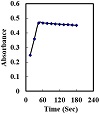
Figure 3: Effect of response time on the absorbance of the proposed optode; conditions: [Hf(IV)] = 100 ng cm−3; pH = 5.0.
To ensure proper color changing, an optode membrane must be reversible. In order to change the color of the Hf(IV) complex, several chemicals were examined. Regenerating reagents such as ethylene diamine, thiourea, SCN, and uric acid were studied. However, there was no additional improvement observed in the optode’s reversibility after partial and lengthy exposure to SCN, EDTA, sulfosalicylic acid, and uric acid. Among the reagents tested, thiourea proved to be the optimal choice, as it had a quick regeneration period of about two minutes. The on use durability of the sensor phase was achieved by subsequently placing the membrane in Hf(IV) solutions and regenerating. After regeneration and for the next Hf(IV) concentration
measurement, the optode should be put in buffer (pH 5.0) for 3–5 min.
The selectivity of the optode membrane was tested, and the BTABPD membrane was examined to determine 100 ng cm−3 of Hf(IV) ions in the presence of some metal ions including Sc3+, Y3+, La3+, Ce3+, Gd3+, Sm3+, Lu3+, Tm3+, Ho3+, Dy3+, Eu3+, Cd2+, Cu2+, Ni2+, Co2+, Pb2+, Bi3+, Al3+, Sn2+, Mn2+, Tl+, Ag+, Zn2+, Fe2+, Cr3+, and Zr4+ ions. The tolerance limit was taken as the concentration causing an error of ±5.0% in the absorbance for Hf(IV) determination [31]. At the applied pH value, no interference was achieved from even 6000-fold excess of the above metal ions. The surprisingly high selectivity of the BTABPD membrane for Hf(IV) ions over other cations examined most probably arises from the strong tendency of the complexation BTABPD for Hf(IV) ions.
Figure 1 shows the absorption spectra of BTABPD and the APASA-Hf(IV) complex. This gives us important information about the wavelengths at which they absorb. Notably, the peak absorption of BTABPD is at 490 nm, while the BTABPD-Hf(IV) complex has a very clear absorption band at 616 nm. To ascertain the stoichiometry of the BTABPD-Hf(IV) complex, two methods, namely, the molar ratio and
Job’s methods, were employed.
The molar ratio method showed that the best absorption happened at a ratio of 2.0 : 1.0 [BTABPD : Hf(IV)], which shows the formation of 2 : 1 complex. Similarly, Job’s method yielded a mole ratio of 0.33, further substantiating the formation of a 2 : 1 stoichiometric ratio in the BTABPD-Hf(IV) complex. These findings, combined with the infrared (IR) spectra of BTABPD and the BTABPD-Hf(IV), provided the basis for proposing the structural representation of the complex as depicted in Scheme 2.
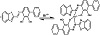
Scheme 2: Representative chemical structure of BTABPD-Hf(IV) complex.
The calibration curve represented that the BTABPD membrane system obeys Beer’s law in the dynamic range of 5.0–185 ng Hf(IV) per cm−3 in the measured samples. For a more accurate analysis, Ringbom optimum concentration range was found to be 15–170 ng Hg2+ per cm−3 in the measured phase. The linear regression equation was found as A = 0.468 C (ng cm−3) + 0.005 (r = 0.9985). The molar absorptivity was evaluated to be 8.36 × 106 L mol−1 cm−1 at 616 nm, whereas Sandell sensitivity was 0.021 ng cm−2. The standard deviations of the absorbance measurements were evaluated from a series of 13 blank solutions. The limits of quantification (K = 10) and of detection (K = 3) of the procedure were illustrated according to the IUPAC definitions [39], C1 = KSo/s where C1 is the limit of detection, K is the constant related to the confidence interval, s is the slope of the standard curve, and So is the standard error of blank. The limits of quantification and of detection limits are found to be 4.95 and 1.58 ng cm−3, respectively.
Reproducibility and repeatability of the optode are two important characteristics. Both parameters were studied in this study. Reproducibility was obtained by determining 100 ng cm−3 of Hf4+ (six replicate determinations). Coefficient variation of the responses between the membranes was 2.15%.
To evaluate the discrepancies in the response for successive runs using a single optode, the repeatability was calculated by performing seven determinations with the same Hf(IV) standard solution. The coefficient of variation of optode response for 100 ng cm−3 Hf(IV) was 1.9%. The mean absorbance values with the standard deviation were found to be 0.382 ± 0.009 (n = 6, 100 ng cm−3 Hf(IV) ion) and 0.015 ± 0.014 (n = 6, 100 ng cm−3 thiourea solution). For the evaluation of differences in responses of individual optodes, four membranes were prepared from the same mixture.
A buffer with pH 5.0 was added to the cuvette which held the membrane to detect the optode lifetime. The absorbance at λmax of 616 nm was measured for around 10 h. It was observed that there was no significant loss of BTABPD during this time. The experiment revealed that the optode remained stable and did not leak when exposed to light. Furthermore, there was no change in absorbance throughout the experiment. However, the prepared membranes were kept under water, when not in use, to prevent them from drying out. Moreover, the stability of the optode response was investigated over 5 weeks under ambient conditions, representing that the optode was stable over this period.
Samples of minerals, tap, river, and waste water were subjected to recovery examinations using the spike approach. Each sample was measured three times. Table 1 shows that Hf(IV) can be recovered with high accuracy from solutions with a concentration ranging between 98.25% and 103.75%. The results obtained using the spike approach were compared with those obtained using the ICP-AES approach, and they were found to be in agreement [40].
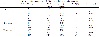
Table 1: Determination of Hf(IV) ion in spiked four water samples.
The process was applied to recover additional amounts of Hf(IV) from four actual water samples to check its accuracy. Table 1 summarizes the recovery outcomes. It shows that the suggested technique can be used to calculate Hf(IV) in various matrices. The applicability of the presented procedure to determine Hf(IV) in real soil samples was tested. The reliability of the method was confirmed by conducting six replicate measurements using the same optode, and the results obtained were found to be satisfactory, as summarized in Table 2. In order to validate the proposed method, spiked real sample was tested and analyzed to assess its performance in real-world scenarios. The accuracy and precision of the proposed procedure were evaluated by comparing the F-test (for precision) and t-value (for accuracy) with the ICP-AES method. The mean values achieved from the proposed procedure were within the acceptable range of Student’s t-test and F-test at a 95% confidence level, considering five degrees of freedom [41]. The calculated values recorded in Table 2 did not exceed the theoretical values, representing good agreement between the results achieved by the proposed and the ICP-AES methods.
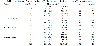
Table 2: Application of the proposed and ICP-AES methods to the determination of Hf in real samples.
The presented procedure was also used to determine Hf(IV) in a new copper-based alloy and zirconium oxides, as a real sample. In this sample, Hf content is lower than the practical detection limit of ICP-AES without preconcentration, so that it cannot be analyzed by direct ICP-AES. This sample was analyzed by the coupling of solid phase extraction to ICP-AES. The results obtained are recorded at Table 2. The t-test showed that there was not any significant difference between the real certified amount and measured Hf(IV). Thus, the proposed membrane can be successfully applied for the determination of Hf(IV) in various real matrices.
The performance of the presented procedure was assessed by the evaluation of the t-value (for accuracy) and F-test (for precision) compared with ICP-AES method. The mean values were achieved in Student’s t- and F-tests at 95% confidence limits for five degrees of freedom [41]. The results indicated that the calculated values (Table 2) did not exceed the theoretical values. A wider range of determination, more stability, higher accuracy, and less time consuming show the advantage of the presented procedure over other ones.
For the determination of low content of hafnium, the presented procedure is highly sensitive and has excellent selectivity. This fact is further confirmed by analyzing various samples of different compositions. The presented procedure was applied successfully to determine hafnium in the terbium-base alloy (72% Tb(III), 9.5% Hf(IV), and 18.5% Al(III)). The result obtained was 9.55 ± 0.11% (RSD 0.76), which confirms the validity of the presented procedure.
The characteristics of the proposed hafnium optode were compared with those of other techniques for measuring hafnium which have been previously published in the literature
(as shown in Table 3). The suggested optode’s linear range and detection limit are acceptable when compared to those reported in previous studies. However, after reviewing the literature, it was found that no prior research has been conducted on an optical sensor membrane with chip reagents for the measurement of Hf(IV) ions in solutions.
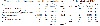
Table 3: Comparison of the proposed optode with other spectrophotometric methods for determination of Hf(IV).
The optode process described in this study is a simple and affordable way to measure Hf(IV) ions. The optode has a long lifespan, is easy to make, and can be regenerated quickly with thiourea. With a high degree of selectivity for Hf(IV) over other lanthanide and transition metal ions, the optode response is reproducible. Unlike regular optical fibers, this optode does not require solvent extraction and can compete favorably. In the study, the optode was used to measure Hf(IV) in different types of water samples, including spiked samples.
The authors are grateful to the Universities of Umm Al-Qura and Benha for the provision of the financial support.
The authors declare that they have no conflict of interest.
- J. R. Rumble, CRC Handbook of Chemistry and Physics, CRC Press, Boca Raton, FL, 87th ed., 2017.
- J. D. Lee, Concise Inorganic Chemistry, Chapman & Hall, London, 5th ed., 1996.
- T. Miura, H. Matsue, and T. Kuroiwa, Instrumental neutron
activation analysis of hafnium in zirconium metal using internal standardization, J Radioanal Nucl Chem, 282 (2009), 49–52.
- T. N. S. Sales, B. Bosch-Santos, M. Saiki, et al., Low temperature synthesis of pure and Fe-doped HfSiO4: Determination of Si and Fe fractions by neutron activation analysis, Rad Phys Chem, 155 (2019), 287–290.
- M. Smolik, H. Polkowska-Motrenko, Z. Hubicki, A. Jakóbik-Kolon, and B. Danko, Determination of hafnium at the 10−4% level (relative to zirconium content) using neutron activation analysis, inductively coupled plasma mass spectrometry and inductively coupled plasma atomic emission spectrometry, Anal Chim Acta, 806 (2014), 97–100.
- N. Y. Varkentin, E. G. Vinokurov, O. A. Karavaeva, and U. V. Bortnikova, X-ray fluorescence determination of small quantities of hafnium in nuclear pure zirconium-based materials, Inorg Mater, 58 (2022), 1415–1421.
- R. Lin, J. Lin, K. Zong, et al., Determination of the isotopic composition of an enriched hafnium spike by MC-ICP-MS using a regression model, Geostand Geoanal Res, 44 (2020), 753–762.
- X. J. Yang and C. Pin, Determination of niobium, tantalum,
zirconium and hafnium in geological materials by extraction chromatography and inductively coupled plasma mass spectrometry, Anal Chim Acta, 458 (2002), 375–386.
- S. Tong, J. Meija, L. Zhou, Z. Mester, and L. Yang, Determination
of the isotopic composition of hafnium using MC-ICPMS, Metrologia, 56 (2019), article no. 044008.
- C. C. Clowers and J. C. Guyon, Spectrophotometric determination of hafnium as reduced molybdosulfatohafnic acid, Anal Chem, 41 (1969), 1140–1142.
- S. Oszwałdowski and J. Jakubowska, Simultaneous determination of zirconium and hafnium as ternary complexes with 5-Br-PADAP and fluoride using solid-phase extraction and reversed-phase liquid chromatography, Talanta, 60 (2003), 643–652.
- T. A. Lasheen, G. M. Hussein, Y. M. Khawassek, and M. F. Cheira, Spectrophotometric determination of zirconium (IV) and hafnium (IV) with pyrazolo (1, 5-a) quinazolin-6-one derivative reagent, Anal Chem Indian J, 12 (2013), 368–376.
- B. Subrahmanyam and M. C. Eshwar, Rapid extractive spectrophotometric determination of hafnium(IV) with 4-(2-pyridylazo)resorcinol, Anal Chem, 47 (1975), 1692–1693.
- Y. K. Agrawal and U. Dayal, Spectrophotometric determination of hafnium as a mixed-ligand complex with N-p-tolyl-p-methoxybenzohydroxamic acid and xylenol orange, Analyst, 109 (1984), 1565–1567.
- Z. Marczenko, Spectrophotometric Determination of Elements, John Wiley & Sons, New York, 1976.
- S. B. Savvin, Arsenazo III, Atom Izdat, Moscow, 1966.
- W. Mäntele and E. Deniz, UV-VIS absorption spectroscopy:
Lambert-Beer reloaded, Spectrochim Acta A Mol Biomol Spectrosc, 173 (2017), 965–968.
- K. L. Cheng, Determination of zirconium and hafnium with xylenol orange and methylthymol blue, Anal Chim Acta, 28 (1963), 41–53.
- B. Subrahmanyam and M. C. Eshwar, Spectrophotometric determination of hafnium(IV) with 1-(2-pyridylazo)-2-naphthol, Mikrochim Acta, 66 (1976), 585–590.
- S. Kalyanaraman and T. Fukasawa, Spectrophotometric determination of zirconium and hafnium with 4-(2-pyridylazo)resorcinol, Anal Chem, 55 (1983), 2239–2241.
- H.-H. Perkampus, UV-VIS Spectroscopy and Its Applications, Springer-Verlag, Berlin, 1992.
- A. Premadas, Cation exchange chromatographic group separation and ICP-AES determination of rare earth elements and yttrium in refractory minerals zircon, ilmenite, rutile, columbite-tantalite, garnet, and sillimanite, At Spectrosc, 24 (2003), 149–158.
- J. Zhao, Y. Sui, X. Peng, G. Sun, and Y. Cui, A new diphosphonic acid extractant N,N-n-octylamine di(methylene phenylphosphinic acid) for extraction and separation of zirconium and hafnium in hydrochloric acid, J Radioanal Nucl Chem, 324 (2020), 339–348.
- T. Yang, G.-X. Sun, Y. Qian, J.-F. Ma, and Y.-L. Li, Separation of hafnium from zirconium in hydrochloric acid solution with di(2-ethylhexyl)phosphoric acid by solvent extraction, Nucl Sci Tech, 30 (2019), article no. 22.
- H. Onishi, Photometric Determination of Trace Metals. Part IIB: Individual Metals, Magnesium to Zirconium, John Wiley & Sons, New York, 1989.
- S. M. Hasany, Habib-Ur-Rehman, A. Rashid, and F. Rashid, Elemental analysis of special materials by X-ray fluorescence spectrometry, J Radioanal Nucl Chem, 133 (1989), 259–270.
- S. J. Hill, ed., Inductively Coupled Plasma Spectrometry and its Applications, Blackwell Publishing, Oxford, UK, 2007.
- M. West, A. T. Ellis, P. J. Potts, et al., Atomic spectrometry update—X-ray fluorescence spectrometry, J Anal At Spectrom, 27 (2012), 1603–1644.
- W. Simon, Technology and application of chemical sensors and biosensors-Quo-Vadis, Chimia, 44 (1990), 395–399.
- W. R. Seitz and M. J. Sepaniak, Chemical sensors based on immobilized indicators and fiber optics, CRC Crit Rev Anal Chem, 19 (1988), 135–173.
- L. K. Chau and M. D. Porter, Optical sensor for calcium: performance,
structure, and reactivity of calcichrome immobilized at an anionic polymer film, Anal Chem, 62 (1990), 1964–1971.
- A. S. Amin, The surfactant-sensitized analytical reaction of niobium with some thiazolylazo compounds, Microchem J, 65 (2000), 261–267.
- A. S. Amin, Cloud-point extraction and spectrophotometric
determination of trace quantities of bismuth in environmental water and biological samples, Spect Lett, 44 (2011), 424–431.
- A. S. Amin and Z. Al-Malah, Utility of dispersive liquid-liquid microextraction based on ionic liquid for spectrophotometric determination of titanium in environmental samples, J Ind Environ Chem, 1 (2017), 22–30.
- A. S. Amin, Utility of cloud-point preconcentration and spectrophotometry for determination of trace amounts of palladium(II) and their analytical applications, Arab J Chem, 9 (2016), S326–S333.
- H. T. S. Britton, Hydrogen Ions, Chapman and Hall, London, 4th ed., 1952.
- A. Safavi and M. Bagheri, Design and characteristics of a mercury (II) optode based on immobilization of dithizone on a triacetylcellulose membrane, Sens Actuators B Chem, 99 (2004), 608–612.
- B. Kuswandi and R. Narayanaswamy, Characterisation of a Hg(II) ion optrode based on Nafion-1-(2-thiazolylazo)-2-naphthol composite thin films, J Environ Monit, 1 (1999), 109–114.
- International Union of Pure and Applied Chemistry, Nomenclature, symbols, units and their usage in spectrochemical analysis—I. General atomic emission spectroscopy analytical chemistry division, Spectrochim Acta Part B At Spectrosc, 33 (1978), 219–239.
- H. Karami, M. F. Mousavi, Y. Yamini, and M. Shamsipur, Online solid phase extraction and simultaneous determination of hafnium and zirconium by ICP—atomic emission spectroscopy, Microchim Acta, 154 (2006), 221–228.
- J. N. Miller and J. C. Miller, Miller Statistics and Chemometrics for Analytical Chemistry, Prentice-Hall, England, 5th ed., 2005.
- A. Boveiri Monji, H. Yousefnia, M. Haji Hosseini, and S. Zolghadri, A green method for selective spectrophotometric determination of hafnium(IV) with aqueous extract of ficus carica tree leaves, Int J Chem Mol Eng, 12 (2018), 149–152.
- W. A. Dupraw, Simple spectrophotometric method for determination of zirconium or hafnium in selected molybdenum-base alloys, Talanta, 19 (1972), 807–810.
- S. Kalyanaraman, A. Sugiyama, and T. Fukasawa, Extraction-spectrophotometric determination of zirconium or hafnium with 1-(2-thiazolylazo)-2-naphthol, Analyst, 110 (1985), 213–214.
Copyright © 2024 Abdullah A. A. Sari et al. This is an open access article distributed under the terms of the Creative Commons Attribution License, which permits unrestricted use, distribution, and reproduction in any medium, provided the original work is properly cited.