Vol. 6 (2023), Article ID 246150, 5 pages
Research Article
A Rhenium(I)-Diselenoether Compound Significantly Decreased the Viral Load of SARS-CoV-2 in Infected Pulmonary Cells
Philippe Collery,1 Sunali Khanna,2 Pascal Grondin,3 Guillaume Fichet,3 and Didier Desmaële4
1Society for the Coordination of Therapeutic Researches, 20220 Algajola, France
2Nair Hospital Dental College, Mumbai, Maharashtra 400008, India
3Oncodesign Services, 21000 Dijon, France
4Galien Institute, University of Paris-Saclay, 91190 Orsay, France
Received 7 December 2022; Revised 2 March 2023; Accepted 2 March 2023; Published 27 April 2023
Philippe Collery, Sunali Khanna, Pascal Grondin, Guillaume Fichet, and Didier Desmaële, A Rhenium(I)-Diselenoether Compound Significantly Decreased the Viral Load of SARS-CoV-2 in Infected Pulmonary Cells, Journal of Transition Metal Complexes, 6 (2023), art246150. doi:10.32371/jtmc/246150
Rhenium(I) tricarbonyl complexes inhibited the 3-chymotrypsin-like protease (3CLpro), a cathepsin cysteine protease, which is one of the main proteases of the severe acute respiratory
syndrome—coronavirus 2 (SARS-CoV-2 virus), but the antiviral effect was not studied. On the other hand, a rhenium(I)-diselenoether complex (Re-diSe), which is a Re(I)tricarbonyl compound with a diselenide ligand, decreased the production of cysteine proteases B and S in cancer and normal cells at doses that did not affect the viability of the normal cells. Objective. To study the effect of Re-diSe on the viral load of SARS-CoV-2 in infected Calu-3 pulmonary human epithelial cells in culture. Methods. Cells were infected with SARS-CoV-2 (European original origin) with one multiplication of infection (MOI 0.01). The viral load was assayed in the supernatant by RT-qPCR after an exposure time of 120 h in cells treated at different doses (1, 5, 10, and 20 μM) and in non-treated cells (controls). In parallel, viability of the cells was assayed with Cell Titer Glo technique. Results. A significant decrease of the viral load was observed at doses of 10 and 20 μM without any cytotoxity. Discussion. Further studies are required to correlate these effects with the activities of host and virus cysteine proteases, but Re-diSe is already a promising drug for the treatment of Covid19.
rhenium (Re); selenium (Se); SARS-CoV-2; viral infection; cathepsins; cysteine proteases
Karges et al. demonstrated that rhenium(I) tricarbonyl complexes inhibited the 3-chymotrypsin-like protease (3CLpro), a cathepsin cysteine protease, which is one of the main proteases of the severe acute respiratory syndrome—coronavirus 2 (SARS-CoV-2 virus), but the antiviral effect was not studied [1]. Metal-based complexes have been proved as able to inhibit cathepsins cysteine proteases [2,3,4,5]. Proteases play a critical role in the process of SARS-CoV-2 entry into the host cells, in viral RNA replication and translation [6,7]. There are two virus cysteine proteases, the Papain-Like cysteine protease (PLpro) and the 3C-Like cysteine protease (3CLpro), also called main protease (MPro). The host proteases are two cell surface serine proteases, a furin protease and the TransMembrane Protease Serine (TMPRSS2) and two cysteine proteases, cathepsin L and B. Inhibitors of these proteases have been suggested as promising therapeutic options for the treatment of Covid19 infection [8,9,10,11,12,13,14,15], which is the infection due to the SARS-CoV-2 virus. We have reported that a rhenium(I)-diselenoether complex (Re-diSe, Figure 1) formed by a Re(I)tricarbonyl core chelated with a bidentate diselenide ligand (two atoms of selenium), decreased the production of cysteine proteases B and S in cancer and normal cells at doses that did not affect the viability of the normal cells [16]. Due to the properties of Re-diSe to decrease the production of cathepsins cysteine proteases it is expected that it could be active against the Covid19 infection.
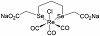
Figure 1: Chemical structure of the Re-diSe complex.
Our aim was thus to investigate the effects of Re-diSe on the viral load of SARS-CoV-2 in infected Calu-3 pulmonary epithelial cells in culture.
The synthesis of the Re-diSe drug was performed as previously reported [17]. The full physico-chemical characterization of the Re complex, was achieved and reported in the initial publication by Kermagoret et al. [18]. This included elemental analysis to confirm purity and X-Ray crystal structure of a related complex definitively establishing the chemical structure. Since that first report, the new batches of complex were routinely characterized by 1H NMR, ESI mass spectrum and IR spectroscopy. A quality control was also performed by LC-UV-HRMS at 100 μg/mL, and the HRMS analysis was performed using LTQ orbitrap XL.
In vitro amplification of pulmonary human epithelial cells Calu-3 (HTB-55™ from American Tissue Culture Collection) was performed for seeding 96 well plates. Cells were grown as monolayer at 37 °C in a humidified atmosphere (5% CO2, 95% air) into the cell culture medium (MEM + 1% pyruvate + 1% glutamine + 10% fetal bovine serum).
Cells are adherent to plastic flasks. For cell passaging, cells were detached from the culture flask by a 20 min treatment with trypsin-versene and neutralized by addition of
complete culture medium. Before seeding, the cells were counted and their viability was assessed by using Vi-cell counter.
The virus strain (SARS-CoV-2 initial European isolate) was supplied through the European Virus Archive – Global (EVAg) platform (https://www.european-virus-archive.com; reference SARS-CoV-2 strain Slovakia/SK-BMC5/2020).
Cell plates were infected with SARS-CoV-2 virus, with one multiplication of infection (MOI: 0.01), corresponding to 100.000 infectious particles per well plate, from the viral stock assaying 1.500.000 pfu/mL.
Re-diSe was added simultaneously with the SARS-CoV-2 virus in the medium of culture and 4 concentrations (20, 10, 5, and 1 μM) were tested for an exposure time of 5 days (120 h). Controls corresponded to Calu-3 cells infected by virus without any treatment. 120 h post-infection supernatant was collected to assay viral load by RT-qPCR.
Quantification of viral load by RT-qPCR has been done from cell supernatant using viral ORF1ab gene. Extraction of viral RNA has been performed using the Macherey Nagel NucleoSpin 96 RNA, 96-well kit for RNA purification (ref. #740709.4). RNAs has been frozen at −80 °C until qRTPCR. RT was performed with the High Capacity cDNA Reverse Transcription Kit from Applied Biosystem (ref. #4368813). cDNA quantification by quantitative PCR was performed with primers conditions targeting ORF1ab gene. Amplifications were performed using a QuantStudio 7 Flex from Applied Biosystem and adjoining software.
|
Primers and probes |
|
Name | Sequences (5′-3′) |
|
ORF1ab gene/nCoV | |
|
ORF1ab_Fw | CCGCAAGGTTCTTCTTCGTAAG |
|
ORF1ab_Rv | TGCTATGTTTAGTGTTCCAGTTTTC |
|
In parallel, to determine the cytotoxic effect of Re-diSe, the 4 concentrations of Re-diSe were incubated on Calu-3 cells for 120 h without virus, and cell viability assay was done with CellTiterGlo technique.
The statistical analysis was performed using GRAPH PAD (T-test classic, non-paired data) on triplicate experiments (n = 3). Results were considered statistically significant when P < .05.
The good quality of the synthesis was verified corresponding to the already described structure of the Re-diSe compound with a degree of purity of 80%.
The schematic structure is depicted in Figure 1.
No toxicity was observed on non-infected pulmonary cells exposed for 120 h even at the highest dose of 20 μMRe-diSe with a cell viability of 100%.
There was a significant decrease on the % of infected cells in cells treated at doses of 10 and 20 μM of Re-diSe by comparison with non-treated infected cells. At the dose of 20 μM, the Re-diSe compound prevented the infection in 60% of the cells while 100% of the untreated cells were infected by the SARS-CoV-2 virus (P = .0033). The results are presented as Figure 2.
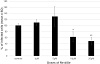
Figure 2: Dose-effect of Re-diSe on the % of infected cells (*P < .05, **P = .0033).
SARS-CoV-2 is an enveloped, single-stranded positive-sense RNA virus with the largest known genome size [19]. The best treatment of the pandemic Covid19 infection is preventive through the vaccinations. Still now, they have not been able to definitively stop it. The main target of vaccines, but also of monoclonal antibodies, is the spike protein (S).
Variants of the spike protein may decrease the binding affinity of monoclonal antibodies reducing their efficiency and also decrease the efficacy of the vaccines.
The architecture of the proteases is conserved among all coronavirus (CoVs). Therefore, mutations do not affect them. However, mutations affect the mode of entry of the virus.
The Omicron SARS-CoV-2 variant could be more dependent on cathepsin L than TMPRSS2 for entry into cell, while during the initial phase of the COVID19 pandemic TMPRSS2 was the most important host factor mediating SARS-CoV-2 entry [20].
TMPRSS2 is widely expressed in epithelial cells of the respiratory, gastrointestinal, and urogenital tract [21]. Inhibitors of the serine protease TMPRSS2 are camostat mesylate and nafamostat mesylate. They are especially used to prevent the pulmonary complications [22,23]. However, a single treatment by the TMPRSS2 inhibitor camostat mesylate did not affect time to clinical improvement, progression to intensive care unit admission, or mortality in a randomized clinical trial [24].
It has been shown that SARS-CoV-2 induced a pro-oxidative status with an excessive use of cysteine in viral protein synthesis and to endoplasmic reticulum (ER) stress pathway activation [25].
There are two virus cysteine proteases involved in the entry of the SARS-CoV-2 in host cells, the papain-like protease (PLpro), the 3C-Like cysteine protease (3CLpro), also called main protease (Mpro). The host proteases involved into the entry of the virus are cathepsins B and L. Another cysteine protease, cathepsin C, is involved in the alveolar lung damages through the activation of immune cell-associated serine proteases such as proinflammatory neutrophil elastase-related serine proteases (elastase, proteinase 3, cathepsin G, and NSP4) by the removal of their N-terminal dipropeptide [26].
Inhibitors of PLpro
Inhibitors of PLpro are under investigation [27]. Metallodrugs are among the candidates, notably gold complexes [28].
Inhibitors of cathepsin L
It has been shown that the cathepsin L cleavage site was conserved in envelope proteins of different families of viruses such as SARS-CoV and Ebola, Hendra, and Nipah viruses, and inhibition of cathepsin L could led to inhibition of entry of these viruses into host cells [29].
Inhibitors of Mpro
It is through its binding with a cysteine in the active site of this main cysteine protease of SARS-CoV-2 that Re-tricarbonyl compounds were able to inhibit its activity [1].
Several inhibitors of MPro have been described, as for example Bepridil® which is a calcium channel blocker used to treat cardiovascular patients with chronic stable anginapectoris [30], Ebselen®, an organoselenium compound [31,32], Disulfiram® [33] which is an FDA-approved drug for the treatment of alcohol dependence, and Carmofur® which is an approved antineoplastic drug, derived from 5-fluorouracil (5-FU) [34], but these drugs have not been tested in randomized clinical trials in Covid19 infected patients.
Nirmatrelvir/ritonavir (Paxlovid®) received the FDA and EMA agreements as antiviral drugs against SARS-CoV-2 Mpro [35]. In this combination, nirmatrelvir is the inhibitor of the protease, but ritonavir is added as a cytochrome P450 inhibitor to increase the plasma concentrations of nirmatrelvir. Therefore, interactions with many drugs will occur, resulting in many contraindications. Moreover, the indications are limited to patients with a high risk of evolution towards a severe Covid infection and often these patients are already receiving these contraindicated common drugs. Finally the treatment must begin very early from the first signs of infection and patients must not need an oxygen therapy treatment. A great advantage is nevertheless the oral administration and this was taken into account for an early access.
Dual inhibitors of both MPro and cathepsin L are under investigation [36], as well as the combination of cysteine
and serine protease inhibitors [37].
Cathepsin cysteine proteases are targets of metal transition-based drugs [4], by substitution with the cysteine thiolate at the enzyme active site.
It is known since 2006 that Re complexes could inhibit cysteine protease B [38] and the mechanism of action was attributed to a nucleophilic displacement with the highly nucleophilic cysteine residue in the active site of the cathepsin. It was already suggested in 2004 that metal-conjugated complexes could inhibit the 3C-Like cysteine protease [2], but it is only recently that Karges and al. showed for the first time that Re(I) tricarbonyl complexes were able to inhibit the enzymatic activity of the main protease MPro [1]. They identified a (CO)3Re-bipyridine complex that bound covalently to Cys145 amino acid within the catalytic active site of the enzyme through a metal-cysteine bond.
Docking studies may be helpful to identify the binding of metal complexes with the targeted proteases [39].
Rhenium(I)-diselenoether is a Re(I)tricarbonyl-diselenide compound (Re-diSe), able to decrease the production of cathepsins cysteine proteases B and S in cancer cells [16]. The selenium (Se) component in this Re-diSe complex may provide additional properties, as Se compounds are under investigation against both cancer and viral infections [40,41]. Changes in redox homeostasis in infected cells are one of the key events that is linked to infection with respiratory viruses and to inflammation and subsequent tissue damage [42]. There is an overproduction of reactive oxygen species (ROS) and reactive nitrogen species (RNS) induced by the virus and many intracellular signaling pathways exploited by viruses for their own replication are regulated by the oxidoreductive (redox) state of the host cell [43,44]. An antioxidant treatment reduced virus production, reduced the viral positive-to-negative strand RNA ratio, and resulted in the accumulation of uncapped positive-sense viral RNAs [45].
Re-diSe also has anti-oxidant properties, decreasing the production of reactive oxygen species (ROS) [46] and therefore could decrease the oxidative stress induced by SARS-CoV-2. In contrast, silver nanoparticles showed antiviral effects inhibiting extracellular SARS-CoV-2 at concentrations ranging between 1 and 10 ppm while cytotoxic effect was observed at concentrations of 20 ppm and above, but with an increased production of free radicals and reactive oxygen species (ROS) [47,48]. However, the silver nanoparticles cleaved the disulfide bonds of the spike and ACE2 proteins of the virus to affect the viral infectivity.
We demonstrated that the Re-diSe compound significantly decreased the viral load of SARS-CoV-2 virus in Calu-3 epithelial pulmonary infected cells in culture without affecting the viability of the normal pulmonary cells. It will be necessary to determine in further studies if it is correlated with the inhibition of cysteine proteases of the host and of the virus, as expected. Physiological levels of cysteine proteases are also essential for regulating the functions of immune cells and the doses should be managed to respect their activities.
Philippe Collery was the initiator of the study. Sunaly Khanna was involved in the discussion part of the manuscript. Pascal Grondin and Guillaume Fichet performed both the quality control by LC-UV-HRMS and the experiments in Oncodesign laboratory and Didier Desmaële synthesized the Re-diSe drug.
Funding was provided by the Society for the Coordination of Therapeutic Researches.
Philippe Collery is manager of the Society for the Coordination of Therapeutic Researches which is owner of the Intellectual Properties of the patent on “Rhenium Complexes and their Pharmaceutical Use”.
- J. Karges, M. Kalaj, M. Gembicky, and S. M. Cohen, ReI tricarbonyl complexes as coordinate covalent inhibitors for the SARS-CoV-2 main cysteine protease, Angew Chem Int Ed Engl, 60 (2021), 10716–10723.
- J. T. Hsu, C. J. Kuo, H. P. Hsieh, et al., Evaluation of metal-conjugated
compounds as inhibitors of 3CL protease of SARS-CoV, FEBS Lett, 574 (2004), 116–120.
- M. Huisman, J. P. Kodanko, K. Arora, et al., Affinity-enhanced luminescent Re(I)- and Ru(II)-based inhibitors of the cysteine protease cathepsin L, Inorg Chem, 57 (2018), 7881–7891.
- S. P. Fricker, Cysteine proteases as targets for metal-based drugs, Metallomics, 2 (2010), 366–377.
- H. M. Abd El-Lateef, T. El-Dabea, M. M. Khalaf, and A. M. Abu-Dief, Development of metal complexes for treatment of coronaviruses, Int J Mol Sci, 23 (2022), 6418.
- M. Kawase, K. Shirato, L. van der Hoek, F. Taguchi, and S. Matsuyama, Simultaneous treatment of human bronchial epithelial cells with serine and cysteine protease inhibitors prevents severe acute respiratory syndrome coronavirus entry, J Virol, 86 (2012), 6537–6545.
- V. Thiel, K. A. Ivanov, A. Putics, et al., Mechanisms and enzymes involved in SARS coronavirus genome expression, J Gen Virol, 84 (2003), 2305–2315.
- Z. Jin, X. Du, Y. Xu, et al., Structure of mpro from SARS-CoV-2 and discovery of its inhibitors, Nature, 582 (2020), 289–293.
- V. Anirudhan, H. Lee, H. Cheng, L. Cooper, and L. Rong, Targeting SARS-CoV-2 viral proteases as a therapeutic strategy to treat COVID-19, J Med Virol, 93 (2021), 2722–2734.
- K. Brix, Host cell proteases: Cathepsins, in Activation of Viruses by Host Proteases, E. Böttcher-Friebertshäuser, W. Garten, and H. Klenk, eds., Springer, Cham, 2018, 249–276.
- W. Cui, K. Yang, and H. Yang, Recent progress in the drug development targeting SARS-CoV-2 main protease as treatment for COVID-19, Front Mol Biosci, 7 (2020), 616341.
- B. Goyal and D. Goyal, Targeting the dimerization of the main protease of coronaviruses: A potential broad-spectrum therapeutic strategy, ACS Comb Sci, 22 (2020), 297–305.
- A. Pogorzelska, B. Żołnowska, and R. Bartoszewski, Cysteine cathepsins as a prospective target for anticancer therapies—current progress and prospects, Biochimie, 151 (2018), 85–106.
- A. D. Rathnayake, J. Zheng, Y. Kim, et al., 3C-like protease inhibitors block coronavirus replication in vitro and improve survival in MERS-CoV-infected mice, Sci Transl Med, 12 (2020), eabc5332.
- D. Shin, R. Mukherjee, D. Grewe, et al., Papain-like protease regulates SARS-CoV-2 viral spread and innate immunity, Nature, 587 (2020), 657–662.
- P. Collery, V. Veena, D. Desmaële, A. Harikrishnan, and B. Lakshmi, Effects of rhenium(I)-diselenoether and of its diselenide ligand on the production of cathepsins B and S by MDA-MB231 breast malignant cells, Anticancer Res, 41 (2021), 5997–6001.
- P. Collery, A. Mohsen, A. Kermagoret, et al., Antitumor activity of a rhenium (I)-diselenoether complex in experimental models of human breast cancer, Invest New Drugs, 33 (2015), 848–860.
- A. Kermagoret, G. Morgant, J. d’Angelo, et al., Synthesis, structural characterization and biological activity against several human tumor cell lines of four rhenium(I) diseleno-ethers complexes: Re(CO)3Cl(PhSe(CH2)2SePh), Re(CO)3Cl(PhSe(CH2)3SePh), Re(CO)3Cl(HO2C-CH2Se(CH2)2SeCH2-CO2H) and Re(CO)3Cl(HO2C-CH2Se(CH2)3SeCH2-CO2H), Polyhedron, 30 (2011), 347–353.
- Y. Chen, Q. Liu, and D. Guo, Emerging coronaviruses: Genome structure, replication, and pathogenesis, J Med Virol, 92 (2020), 418–423.
- P. Kumar, K. M. Ratia, J. M. Richner, et al., Dual inhibition of cathepsin L and 3CL-Pro by GC-376 constrains SARS Cov2 infection including omicron variant, preprint.
- T. H. Bugge, T. M. Antalis, and Q. Wu, Type II transmembrane serine proteases, J Biol Chem, 284 (2009), 23177–23181.
- P. Breining, A. L. Frolund, J. F. Hojen, et al., Camostat mesylate against sars-cov-2 and covid-19-rationale, dosing and safety, Basic Clin Pharmacol Toxicol, 128 (2021), 204–212.
- K. Li, D. K. Meyerholz, J. A. Bartlett, and P. B. McCray Jr., The TMPRSS2 inhibitor nafamostat reduces SARS-CoV-2 pulmonary infection in mouse models of COVID-19, mBio, 12 (2021), e0097021.
- J. D. Gunst, N. B. Staerke, M. H. Pahus, et al., Efficacy of the TMPRSS2 inhibitor camostat mesilate in patients hospitalized with Covid-19-a double-blind randomized controlled trial, EClinicalMedicine, 35 (2021), 100849.
- F. Galli, G. Marcantonini, D. Giustarini, et al., How aging and oxidative stress influence the cytopathic and inflammatory effects of SARS-CoV-2 infection: The role of cellular glutathione and cysteine metabolism, Antioxidants (Basel), 11 (2022), 1366.
- B. Korkmaz, A. Lesner, S. Marchand-Adam, C. Moss, and D. E. Jenne, Lung protection by cathepsin C inhibition: A new hope for COVID-19 and ARDS?, J Med Chem, 63 (2020), 13258–13265.
- D. Tian, Y. Liu, C. Liang, et al., An update review of emerging small-molecule therapeutic options for COVID-19, Biomed Pharmacother, 137 (2021), 111313.
- M. Gil-Moles, S. Turck, U. Basu, et al., Metallodrug profiling against SARS-CoV-2 target proteins identifies highly potent inhibitors of the S/ACE2 interaction and the papain-like protease PLpro, Chemistry, 27 (2021), 17928–17940.
- H. A. Elshabrawy, J. Fan, C. S. Haddad, et al., Identification of a broad-spectrum antiviral small molecule against severe acute respiratory syndrome coronavirus and Ebola, Hendra, and Nipah viruses by using a novel high-throughput screening assay, J Virol, 88 (2014), 4353–4365.
- E. C. Vatansever, K. S. Yang, A. K. Drelich, et al., Bepridil is potent against SARS-CoV-2 in vitro, Proc Natl Acad Sci U S A, 118 (2021), e2012201118.
- K. Amporndanai, X. Meng, W. Shang, et al., Inhibition mechanism of SARS-CoV-2 main protease by ebselen and its derivatives, Nat Commun, 12 (2021), 3061.
- L. Y. Sun, C. Chen, J. Su, et al., Ebsulfur and ebselen as highly potent scaffolds for the development of potential SARS-CoV-2 antivirals, Bioorg Chem, 112 (2021), 104889.
- N. Fillmore, S. Bell, C. Shen, et al., Disulfiram use is associated with lower risk of COVID-19: A retrospective cohort study, PLoS One, 16 (2021), e0259061.
- Z. Jin, Y. Zhao, Y. Sun, et al., Structural basis for the inhibition of SARS-CoV-2 main protease by antineoplastic drug carmofur, Nat Struct Mol Biol, 27 (2020), 529–532.
- D. V. Parums, Editorial: Current status of oral antiviral drug treatments for SARS-CoV-2 infection in non-hospitalized patients, Med Sci Monit, 28 (2022), e935952.
- X. R. Ma, Y. R. Alugubelli, Y. Ma, et al., MPI8 is potent against SARS-CoV-2 by inhibiting dually and selectively the SARS-CoV-2 main protease and the host cathepsin L, ChemMedChem, 17 (2022), e202100456.
- P. Padmanabhan, R. Desikan, and N. M. Dixit, Targeting TMPRSS2 and Cathepsin B/L together may be synergistic against SARS-CoV-2 infection, PLoS Comput Biol, 16 (2020), e1008461.
- R. Mosi, I. Baird, J. Cox, et al., Rhenium inhibitors of cathepsin B (ReO(SYS)X (where Y = S, py; X = Cl, Br, SPhOMe-p)): Synthesis and mechanism of inhibition, J Med Chem, 49 (2006), 5262–5272.
- H. M. A. El-Lateef, M. M. Khalaf, M. R. Shehata, and A. M. Abu-Dief, Fabrication, DFT calculation, and molecular docking of two Fe(III) imine chelates as anti-COVID-19 and pharmaceutical drug candidate, Int J Mol Sci, 23 (2022), 3994.
- W. Ali, R. Benedetti, J. Handzlik, C. Zwergel, and C. Battistelli, The innovative potential of selenium-containing agents for fighting cancer and viral infections, Drug Discov Today, 26 (2021), 256–263.
- P. Collery, Strategies for the development of selenium-based anticancer drugs, J Trace Elem Med Biol, 50 (2018), 498–507.
- O. A. Khomich, S. N. Kochetkov, B. Bartosch, and A. V. Ivanov, Redox biology of respiratory viral infections, Viruses, 10 (2018), 392.
- L. Nencioni, R. Sgarbanti, D. Amatore, et al., Intracellular redox signaling as therapeutic target for novel antiviral strategy, Curr Pharm Des, 17 (2011), 3898–3904.
- S. Damiano, C. Sozio, G. La Rosa, and M. Santillo, NOX-dependent signaling dysregulation in severe COVID-19: Clues to effective treatments, Front Cell Infect Microbiol, 10 (2020), 608435.
- R. C. Gullberg, J. Jordan Steel, S. L. Moon, E. Soltani, and B. J. Geiss, Oxidative stress influences positive strand RNA virus genome synthesis and capping, Virology, 475 (2015), 219–229.
- P. Collery, V. Veena, A. Harikrishnan, and D. Desmaele, The rhenium(I)-diselenoether anticancer drug targets ROS, TGF-β1, VEGF-A, and IGF-1 in an in vitro experimental model of triple-negative breast cancers, Invest New Drugs, 37 (2019), 973–983.
- S. S. Jeremiah, K. Miyakawa, T. Morita, Y. Yamaoka, and A. Ryo, Potent antiviral effect of silver nanoparticles on SARS-CoV-2, Biochem Biophys Res Commun, 533 (2020), 195–200.
- N. S. Al-Radadi and A. M. Abu-Dief, Silver nanoparticles (AgNPs) as a metal nano-therapy: possible mechanisms of antiviral action against COVID-19, Inorg Nano-Metal Chem, 2022.
Copyright © 2023 Philippe Collery et al. This is an open access article distributed under the terms of the Creative Commons Attribution License, which permits unrestricted use, distribution, and reproduction in any medium, provided the original work is properly cited.