Vol. 6 (2023), Article ID 246149, 6 pages
Research Article
Formation Equilibria and Thermodynamic Stability of Multinuclear Chelates of Amino Acid and Pyrimidine Base Involving Some Bio-Active Transition Metal Ions
V. P. Shukla1 and Surabhi Sinha2
1Department of Chemistry, Bipin Bihari College, Jhansi 284001, India
2Department of Chemistry, United College of Engineering & Research, Allahabad 211010, India
Received 8 November 2022; Revised 8 January 2023; Accepted 9 January 2023; Published 27 April 2023
V. P. Shukla and Surabhi Sinha, Formation Equilibria and Thermodynamic Stability of Multinuclear Chelates of Amino Acid and Pyrimidine Base Involving Some Bio-Active Transition Metal Ions, Journal of Transition Metal Complexes, 6 (2023), art246149. doi:10.32371/jtmc/246149
The coordination tendency of amino acid (valine) to form heterobinuclear complexes with some bi-positive metal ions (Co(II), Ni(II), Cu(II), Zn(II)) in the presence of pyrimidine base (uracil) has been explored potentiometrically in aqueous medium. Formation constants of the complexes at 37 ± 10 at a constant ionic strength of 0.1 NaNO3 have been evaluated using the SCOGS computer program. Solutions of cobalt, nickel, copper, zinc, and metal ions (0.01 M) were prepared and standardized by EDTA titration. The uracil solution (0.01 M) was prepared by dissolving the ligand into one equivalent of alkali sodium hydroxide, whereas the valine solution (0.01 M) was prepared in double-distilled water only. The speciation curves were finally sketched by running the computer program ORGIN4.0. The overall stability constants of binary, ternary and multinuclear chelates followed the Irving–William order. Titration and speciation distribution curves were found to be favourable for the complexation.
multinuclear chelates; amino acid (valine); pyrimidine base (uracil); SCOGS; ORGIN4.0
The recommended medical procedure for lowering metal toxicity is chelation therapy. The main contribution of chemistry to this area is in the determination of speciation constants as well as coordination models of complexes between chelating agents and metal ions (such as Co, Ni, Cu, Zn, Pb, Cd, etc.) to compare the properties and strength of produced complexes. The number of reported
species in research findings has improved due to computer modelling techniques and possibly a deeper comprehension of solution equilibria for metal-amino acid complexes. In the speciation process using in vivo chelation, it should be taken into account that there is always competition for the coordinating positions between essential and toxic metal ions such as Cu(II), Zn(II), Mn(II), Mg(II), Ir(II) [1,2]. Coordination chemistry in metalloproteins is dominated by amino acids [3,4]. The concentration of metals and amino acids and the thermodynamic processes governing complex formation are all necessary for complex formation. At pH 7, the side chains of valine are nonpolar or positively charged, which restricts their ability to bind cations to bidentate binding through the amine nitrogen and carboxylate oxygen groups. Metal chelates as functional and structural motifs play an important role in the development of a new model of complexes [5]. Mixed ligand and multimetal-multiligand complexes play an important role in biological [6,7,8] processes as exemplified by many instances in which enzymes [9,10] are known to activate metal ions [11,12]. Cobalt, nickel and copper are important trace elements for plants and animals [13] and are involved in ternary complexation. Transition metals are involved in many redox processes requiring electron transfer [14]. In continuation to our studies on mixed chelates [15,16,17,18,19,20,21,22,23,24,25,26], we report herein the speciation equilibria of multimetal-multiligand complexes, their overall formation constants and percentage distribution curves in accordance with the pH variations. The probable solution structures of metal complexes with the said ligands and the order of speciation constants of multinuclear chelates have been discussed.
All the solutions were prepared in double-distilled carbon dioxide-free water. Metal nitrate solutions were standardized by ethylene diamine tetra acetic acid (complexometric titration) [27,28]. A digital pH meter century-model (CP901-S) with glass electrode at 37 ± 1 °C, reading up to 0.01 units, was used for measuring the pH of prepared solutions. The potentiometer was standardized using buffer solutions of 4.0, 7.0 and 9.2 pH. Calvin-Bjerrum pH titration technique as modified by Irving and Rossotti [29,30] was used for the potentiometric determination of proton-ligand and metal-ligand stability constants. Binary M:L1/M:L2 (1:1), ternary M:L1:L2 (1:1:1) and quaternary M1:M2:L1:L2 (1:1:1:1) metal-ligand mixtures of the following composition were prepared for titration, keeping a total volume of 50 mL in each case. The strength of metal and ligand = 0.01 M and ionic strength I = 0.1 M sodium nitrate (NaNO3).
Solution A: 5 mL NaNO3 (1.0 M) + 5 mL HNO3 (0.02 M) + water.
Solution B: 5 mL NaNO3 (1.0 M) + 5 mL HNO3 (0.02 M) + 5 mL L1 (0.01 M) + water.
Solution C: 5 mL NaNO3 (1.0 M) + 5 mL HNO3 (0.02 M) + 5 mL L1 (0.01 M) + 5 mL M1(II) (0.01 M) + water.
Solution D: 5 mL NaNO3 (1.0 M) + 5 mL HNO3 (0.02 M) + 5 mL L1 (0.01 M) + 5 mL M1(II) (0.01 M) + 5 mL L2 (0.01 M) + water.
Solution E: 5 mL NaNO3 (1.0 M) + 5 mL HNO3 (0.02 M) + 5 mL L1 (0.01 M) + 5 mL M1(II) (0.01 M) + 5 mL L2 (0.01 M) + 5 mL M2(II) (0.01 M) + water.
M1(II) and M2(II) are Co/Ni/Cu and Zn, L1 is the primary ligand and L2 is the secondary ligand. The pH meter readings with progressive addition of alkali to the titration mixtures were noted, once the reading gets stabilized. The titration was discontinued at the appearance of turbidity. The pH values were plotted against the volume of NaOH and titration curves were obtained. For the evaluation of stability constants by the SCOGS computer program [31] in a system of the two different metal ions M1 and M2 and two different ligands L1 and L2 in an aqueous medium, speciation may be described according to equilibrium:
pM1 + qM2 + rL1 + sL2 + tOH ⇌ (M1)p(M2)q(L1)r(L2)s(OH)t. |
The overall stability constant (βpqrst) defined as
βpqrst = [(M1)p(M2)q(L1)r(L2)s(OH)t]/[M]p[M2]q[L1]r[L2]s[OH]t |
may be used to calculate the species distribution curves that provide the clues for the formation equilibria of the complexes. The values of the constants were supplied to the computer as input data to obtain the distribution curves of the complexes occurring at different pH. The ionic product of water (kw) and activity coefficient of the hydrogen ion under the experimental conditions were obtained from the literature.
Valine is an aliphatic, nonpolar, hydrophobic amino acid. It contains two potentially ionizable functional groups (COOH and NH2) and behaves as a bidentate ligand. The side chain of valine is highly nonreactive and is thus rarely directly involved in protein function. Particularly hydrophobic amino acids may be engaged in the binding or recognition of lipids and other hydrophobic ligands. The ionization constants’ (pK) values correspond to the ionization of a proton from amine [32] and carboxylic groups.
Much interest has been shown in the chemistry of β-lactam in relation to their useful biological activities in recent years. It is known that antibiotic activity is related to the ability of these compounds to form complexes with metal ions [33].
Uracil (a pyrimidine base) undergoes keto-enol tautomeric shift because of its resonating structures due to amine (NH2) [34] and hydroxyl (OH) substrant.
Proton ionization from uracil in the strongly acidic medium has been reported based on spectroscopic data. Levene, Bass and Simme observed that uracil has two ionizable protons, yet they yield only one pK value, i.e., 9.45, whereas Srivastava obtained its value 9.16 at 30 °C and 0.1 M (NaNO3) ionic strength. Protonation constants for the ligands have been determined by Irving–Rossotti titration technique [35] as presented in Table 1.
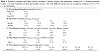
Table 1: Stability constants and other related constants of binary, ternary and quaternary complex of α-ε-diaminocaproate (valine = A) and 5-methyl-2,4-dioxopyrimidine (uracil = B) with different metal ions in an aqueous solution at 37 ± 1 °C, I = 0.1 M NaNO3.
Potentiometric titration curves and formation curves for [Cu(II)-Co(II)-valine-uracil] system are presented in Figures 1 and 2, respectively. pH titration curves clearly show that curve E (quaternary) sets apart from curve D (ternary) at pH ≈ 4.2, indicating complex formation. Multimetal-multiligand complex formation equilibria are found to incorporate quaternary complex species, H2L, HL (valine), HL (uracil), binary, ternary complex species, free metal ions and hydroxo species in the present system. All the protonated ligand species are found to be decreasing with the increase in the pH range ≈ 3.3–8.5, which shows their involvement in the complex formation. The formation of metal-ligand complexes is indicated by
[HL1]− + Cu2+ ⇌ [Cu(II)−L1] + H+, |
[HL2]− + Cu2+ ⇌ [Cu(II)−HL2]+, |
[HL1]− + Co2+ ⇌ [Co(II)−L1] + H+. |
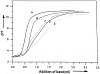
Figure 1: Potentiometric titration curves of 1:1:1:1 Cu(II)-Co(II)-valine-uracil system; (A) acid, (B) valine, (C) Cu(II)-valine, (D) Cu(II)-valine-uracil and (E) Cu(II)-Co(II)-valine-uracil.
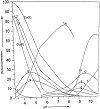
Figure 2: Distribution curves of 1:1:1:1 Cu(II)-Co(II)-valine-uracil system; (1) H2L1, (2) HL1, (3) HL2, (4) Cu(OH)+, (5) Cu(OH)2, (6) Co(OH)+, (7) Co(OH)2, (8) CuL1, (9) CuL2, (10) CoL1, (11) CoL2, (12) CuL1L2, (13) CoL1L2 and (14) CuCoL1L2.
The binary complexes [Cu(II)–L1] and [Cu(II)–L2H]+ are present in the appreciable amount in the pH range ≈ 3.2–9.0. The binary complexation starts in the beginning of titration and the mentioned complexes attain maximum concentration at ≈ 29% and ≈ 19%, respectively, at pH ≈ 4.5. However, with further increase in pH, their concentrations decrease.
Mixed ligand complexes with Cu2+ (aq.) and Co2+ (aq.) are found to be remarkable species in a higher pH range ≈ 5.4–10.2 as follows:
[HL1]− + Cu2+ + [HL2]− ⇌ [Cu(II)−L1−L2H]− + H+, |
[Cu(II)−HL2]+ + [L1H]− ⇌ [Cu(II)−L1−L2H]− + H+, |
[L1H]− + Co2+ + [L2H]− ⇌ [Co(II)−L1−L2H]− + H+. |
For the quaternary system, the species distribution curves indicate the formation of a heterobinuclear complex according to the following equilibrium:
[HL1]− + Cu2+ + [HL2]− + Co2+ ⇌ [Cu(II)−Co(II)L1L2] + 2H+. |
Another form of multinuclear equilibrium can be written as
[Cu(II)−L1−HL2]− + Co2+ ⇌ [Cu(II)−Co(II)−L1−L2] + H+, |
[Co(II)−L1−HL2]− + Cu2+ ⇌ [Co(II)−Cu(II)−L1−L2] + H+. |
The speciation curve shows that there is a concomitant decline in the concentration of Cu2+ and Co2+ aqueous ions with the incline in the concentration of multimetal-multiligand complex species. Quaternary complex species is the predominant species in the present system. The complexation starts from the very beginning and the concentration of multinuclear species increases gradually with the gradual increase in pH attaining a maximum value of ≈ 77% at ≈ 7.5. Metal hydroxo species Co(II)(OH)+ and Co(II)(OH)2 exit in the pH range ≈ 7.4–10.2 involving the following equilibrium:
Co2+ + H2O ⇌ Co(II)(OH)+ + H+, |
Co2+ + 2H2O ⇌ Co(II)(OH)2 + 2H+. |
Titration and speciation curves for [Ni(II)-Zn(II)-valine-uracil] system are presented in Figures 3 and 4, respectively. Multinuclear species, protonated species, H2L, HL (valine), HL (uracil), binary, ternary, free metal ions and hydroxo species exist in a considerable amount throughout the entire pH range. It is clearly evident from the species distribution curves that the protonated ligand species H2L and HL of both ligands are found to be decreasing with increase in pH range ≈ 3.2–7.5. The binary complex species [Ni(II)-valine] and [Zn(II)-valine] exist in a higher pH range ≈ 7.8–9.0, while [Ni(II)-uracil] and [Zn(II)-uracil] species are absent in the system. The complexation equilibria for binary complexes may be as follows:
[HL1]− + Ni2+ ⇌ [Ni(II)−L1] + H+, |
[HL1]− + Zn2+ ⇌ [Zn(II)−L1] + H+. |
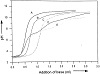
Figure 3: Potentiometric titration curves of 1:1:1:1 Ni(II)-Zn(II)-valine-uracil system; (A) acid, (B) valine, (C) Ni(II)-valine, (D) Ni(II)-valine-uracil and (E) Ni(II)-Zn(II)-valine-uracil.
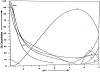
Figure 4: Distribution curves of 1:1:1:1 Ni(II)-Zn(II)-valine-uracil system; (1) H2L1, (2) HL1, (3) HL2, (4) Ni(OH)+, (5) Ni(OH)2, (6) Zn(OH)+, (7) Zn(OH)2, (8) NiL1, (9) NiL2, (10) ZnL1, (11) ZnL2, (12) NiL1L2, (13) ZnL1L2 and (14) NiZnL1L2.
The formation of ternary complex species NiL1L2 and ZnL1L2 occurs in a higher pH range ≈ 8.0–9.0. The concentration of the mixed ligand complex inclines with the increase in pH and attains maximum concentration ≈ 25% and 10%, respectively, according to the following equilibria:
[HL1]− + Ni2+ + [HL2]− ⇌ [Ni(II)−L1−HL2]− + H+, |
[HL1]− + Zn2+ + [HL2]− ⇌ [Zn(II)−L1−HL2]− + H+. |
The alternative step-wise equilibria may be indicated as follows:
[Ni(II)−L1] + [HL2]− ⇌ [Ni(II)−L1−HL2]−, |
[Zn(II)−L1] + [HL2]− ⇌ [Zn(II)−L1−HL2]−. |
For the multimetal-multiligand system, formation curves indicate the complex formation equilibria as follows:
[HL1]− + Ni2+ + [HL2]− + Zn2+ ⇌ [Ni(II)−Zn(II)−L1−L2] + 2H+. |
This may be represented as
[Ni(II)−L1−HL2]− + Zn2+ ⇌ [Ni(II)−Zn(II)−L1−L2] + H+. |
Speciation curves clearly reveal that the concentration of free metal ions continuously falls with the increase in pH, which shows an involvement of free metal ions in the formation of multinuclear complexes. The complexation starts at a very low pH region and the concentration of quaternary complexes with an increase in pH attains maximum value ≈ 85% at pH 7.6. The metal hydroxo species also exists in the system in the pH range ≈ 6.9–9.0 and attains maximum value ≈ 50%:
Zn2+ + 2H2O ⇌ Zn(II)(OH)2 + 2H+. |
The most used technique for determining amino acid-metal binding constants is potentiometric analysis. When combined with the computational power of modelling software, it enables the detection of small species.
Refined values of binary, ternary and quaternary constants are listed in Table 1, which are in good agreement with those in the literature. The overall stability constants of mixed metal-mixed ligand [M1-M2-valine-uracil] systems have been found to follow the following order:
Cu─Ni > Cu─Zn > Cu─Co > Ni─Zn > Ni─Co > Co─Zn. |
Solvated Co2+ ion is water coordinated from six molecules though only tetragonal coordination is feasible as two water molecules are situated at a longer distance. Normally regular octahedral is the preferred configuration of the hydrated nickel ion. However, strong field ligand may enforce tetragonal distortion leading to square planar configuration. The configuration of zinc is regulated by the nature of the ligand bounded which can normally be converted to octahedral from tetrahedral geometry; the metal-ligand complex having the higher value of log β (ligand A) will be the first to attach, which further attaches to ligand B. The uncoordinated sites of ligands will then be occupied by another metal ion (Figure 5).
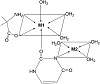
Figure 5: Proposed solution structure of M1(II)-M2(II)-valine-uracil quaternary system.
The authors declare that they have no conflict of interest.
- J. K. Dunnick and B. A. Fowler, Cadmium, in Handbook on Toxicity of Inorganic Compounds, H. G. Seiler, H. Sigel, and A. Sigel, eds., Marcel Dekker, New York, 1988, 156–174.
- A. Catsch and A.-E. Harmuth-Hoene, Pharmacology and therapeutic applications of agents used in heavy metal poisoning, Pharmacol Ther A, 1 (1976), 1–118.
- R. M. Roat-Malone, Bioinorganic Chemistry: A Short Course, John Wiley & Sons, Hoboken, NJ, 2002.
- M. A. Zayed and S. M. Abdallah, Synthesis, characterization and electronic spectra of cefadroxil complexes of d-block elements, Spectrochim Acta A Mol Biomol Spectrosc, 60 (2004), 2215–2224.
- K. D. Karlin, Metalloenzymes, structural motifs, and inorganic models, Science, 261 (1993), 701–708.
- A. Sigel and H. Sigel, eds., Metal Ions in Biological System, vol. 1–38, Marcel Dekker, New York, 1971–2001.
- J. M. Moratal, H. R. Jiménez, J. Castells, J. Salgado, M.-J. Martínez-Ferrer, and A. Donaire, 1H NMR and UV-Vis spectroscopic characterization of sulfonamide complexes of nickel(II)-carbonic anhydrase. Resonance assignments based on NOE effects, J Inorg Biochem, 45 (1992), 231–243.
- K. D. Karlin and Z. Tyeklar, eds., Bioinorganic Chemistry of Copper, Chapman & Hall, New York, 1993.
- S. T. Chow and C. A. Mcauliffe, Transition metal complexes containing tridentate amino acids, in Progress in Inorganic Chemistry, S. J. Lippard, ed., vol. 19, John Wiley & Sons, London, 1975, 51–103.
- H. Irving and R. Williams, Order of stability of metal complexes, Nature, 162 (1948), 746–747.
- L. Hellerman and C. C. Stock, Activation of enzymes: V. The specificity of arginase and the non-enzymatic hydrolysis of guanidino compounds. Activating metal ions and liver arginase, J Biol Chem, 125 (1938), 771–793.
- B. L. Valle and J. E. Coleman, Comprehensive Biochemistry, 8 (1968), 1458.
- D. Gaudin and J. H. Fellman, The biosynthesis of DOPA in albino skin, Biochim Biophys Acta, 141 (1967), 64–70.
- R. Hanna and J. A. Doudna, Metal ions in ribozyme folding and catalysis, Curr Opin Chem Biol, 4 (2000), 166–170.
- D. Bartaria, S. Sinha, and V. Krishna, Formation and stability of heterobinuclear complexes containing Hg II and divalent metal ions with EDTA and CDTA, J Indian Chem Soc, 83 (2006), 198–200.
- D. Bartaria, B. K. Srivastava, and V. Krishna, Equilibrium and synthetic studies of homo- and heterobinuclear complexes of DTPA with ZnII and some transition and alkaline earth metal ions, J Indian Chem Soc, 84 (2007), 1199–1201.
- D. Bartaria, V. P. Shukla, and V. Krishna, Chemical speciation and thermodynamic stability of quaternary mixed chelates of biometals involving lysine, proline and uracil, J Indian Chem Soc, 86 (2009), 9–13.
- P. Chandra, M. Singh, D. Bartaria, and V. Krishna, Complexation behavior and stability of ternary complexes of glutathione and thymine involving HgII, PbII, CdII, ZnII, CoII and NiII metal ions, J Indian Chem Soc, 87 (2010), 955–958.
- D. Bartaria, P. Chandra, M. Singh, and V. Krishna, A comparative study on the interaction of some metal ions with glutamic acid and L-cysteine as primary ligands and thymine as a secondary ligand using potentiometry in aqueous medium, Int J Res Chem Environ, 2 (2012), 45–51.
- V. P. Shukla, S. Sinha, and V. Krishna, Multiple equilibria and chemical distribution of some bio metals with β-amide α-aminosuccinate and α-aminoisoverate as primary ligand and 5-methyl 2,4-dioxopyrimidine as secondary ligand, IOSR J Appl Chem, 4 (2013), 21–26.
- S. Sinha, V. P. Shukla, P. P. Singh, and V. Krishna, Chemical distribution and structure of quaternary metal chelates in aqueous solution involving asparagine and uracil, Chem Sci Trans, 3 (2014), 576–581.
- S. Verma, D. Singh, R. Kumar, B. K. Shukla, and V. Krishna, Equilibrium study and stability constants of mixed ligand complexes of bio-molecules and amino acids with metal ions by potentiometric method, Res J Chem Sci, 5 (2015), 42–48.
- S. Sinha, V. P. Shukla, and V. Krishna, Percentage distribution and structural elucidation of quaternary metal chelates of proline with IMDA and uracil in aqueous medium, Inorg Chem, 11 (2016), 58–64.
- M. Singh, V. Shankar, D. Singh, and V. Krishna, Chelation and stabilization properties of citrulline and uracil with Hg(II) as a heavy metal ion in solution, Chem Sci Trans, 6 (2017), 646–652.
- M. Singh, S. Sinha, and V. Krishna, Computed distribution of
quaternary complexes of Cu(II), Zn(II) Co(II) and Ni(II) with citrulline and tryphtophan as primary ligand and thymine as secondary ligand, Proc Natl Acad Sci India Sect A Phys Sci, 91 (2021), 1–7.
- V. P. Shukla, S. Sinha, and V. Krishna, Complexation behaviour and speciation equilibria of multimetal multiligand complexes of bioactive transition metals involving α – ε – diaminocaproate and 5-methyl-2,4-dioxopyrimidine, Res Rev Electrochem, 12 (2022), 241.
- I. M. Kolthoff, E. B. Sandell, E. J. Meehan, and S. Bruckenstein, Quantitative Chemical Analysis, MacMillan, New York, 4th ed., 1969.
- G. Schwarzenbach and W. Biedermann, Komplexone X. erdalkalikomplexe
von o,o′-dioxyazofarbstoffen, Helvetica Chimica Acta, 31 (1948), 678–687.
- H. Irving and H. S. Rossotti, Methods for computing successive stability constants from experimental formation curves, J Chem Soc, 1953 (1953), 3397–3405.
- H. Irving and H. S. Rossotti, The calculation of formation curves of metal complexes from pH titration curves in mixed solvents, J Chem Soc, 1954 (1954), 2904–2910.
- I. G. Sayce, Computer calculation of equilibrium constants of species present in mixtures of metal ions and complexing agents, Talanta, 15 (1968), 1397–1411.
- A. E. Martell and R. M. Smith, eds., Critical Stability Constants, vol. 1–6, Plenum Press, New York, 1974–1989.
- E. G. Brown, Ring Nitrogen and Key Biomolecules: The Biochemistry of N-Heterocycles, Kluwer Academic Publishers, Boston, 1998.
- L. G. Sillén and A. E. Martell, eds., Stability Constant of Metal-Ion Complexes. Special Publication No. 25, The Chemical Society, London, 1971.
- K. Maskos, Interaction of metal ions with nucleic acids. Interaction of copper(II) with adenosine and its derivatives, Acta Biochim Pol, 25 (1978), 311–323.
Copyright © 2023 V. P. Shukla and Surabhi Sinha. This is an open access article distributed under the terms of the Creative Commons Attribution License, which permits unrestricted use, distribution, and reproduction in any medium, provided the original work is properly cited.